1. Introduction
The use of fossil fuels as a major energy source leads to the accumulation of atmospheric greenhouse gases (GHGs) and ultimately global climate change (Liu et al., 2021; Sonthalia and Kumar, 2021). As an alternative to gasoline, bioethanol, which is produced by the fermentation of plant sources, such as corn or sugarcane, can contribute to carbon reduction (Azhar et al., 2017; Liu et al., 2021). Cassava is a low-cost, high-starch crop grown in tropical and subtropical regions (Li et al., 2017; Sivamani and Baskar, 2018). Cassava is currently used for starch production and alcohol fermentation (Latif and Müller 2014; Li et al., 2017). With the increasing demand for bioethanol, cassava can be used as an alternative source in the production of bioethanol near growing areas, thus making it cost-competitive.
Saccharomyces cerevisiae strains are commonly used in the food industry and laboratories because they are easy to culture and are relatively straightforward in a genetic sense (Zheng et al., 2019). In addition, S. cerevisiae strains have been widely used for bioethanol production because of their high yield (Lee et al., 2017). S. cerevisiae can tolerate various stresses under industrial fermentation conditions, such as low pH, which prevents bacterial contamination (Zheng et al., 2019).
For bioethanol production from starch crops, such as corn and cassava, two processes are required: 1) hydrolysis of starch into glucose by amylase and glucoamylase and 2) fermentation of glucose to ethanol by S. cerevisiae. The two processes can be performed sequentially, which is called separate hydrolysis and fermentation (SHF) (Dahnum et al., 2015). In SHF, the hydrolysis and fermentation processes are performed in different reactors (Lee et al., 2010). Alternatively, these two processes can be performed in one reactor by adding enzymes and yeast cells together; the combined process is called simultaneous saccharification and fermentation (SSF) (Cantarella et al., 2004; Lee et al., 2010; Zhu et al., 2012). Depending on the type of enzyme, yeast cells, raw materials, and fermentation conditions, the fermentation profiles of SHF and SSF can vary greatly (Zhu et al., 2012).
In this study, the commercial wine yeast S. cerevisiae EC1118 was tested for its ability to ferment high-solids cassava (30% w/v). In addition, the SHF and SSF of high-solid cassava were compared to design a suitable fermentation process.
2. Materials and methods
S. cerevisiae EC1118, a commercial wine yeast strain, was used in this study (Jung et al., 2021; Kong et al., 2018). Yeast cells were precultured in YP medium (10 g/L yeast extract and 20 g/L Bacto™ peptone) containing 20 g/L glucose for 24 h at 30°C under aerobic conditions (250 rpm). Precultured yeast cells were collected by centrifugation at 3,314 ×g for 5 min and washed twice with sterile distilled water. The initial cell concentration during fermentation was adjusted to 1.0 of an optical density at 600 nm (OD600).
Peeled and frozen cassava was produced in 2020 and purchased online (World Food, Vietnam). The samples were dried at 60°C for 24 h (10% moisture content), ground, and stored at −80°C until use. For fermentation, distilled water was added to 15 g of cassava powder in a 250-mL flask to make a total volume of 50 mL (30% w/v). The solution was sterilized at 121°C for 15 min. For liquefaction, 5 μL of α-amylase (Liquoflow pHlex DS, Novozymes, Bagsvaerd, Denmark) was added to the cassava solution, and the flask was incubated at 80°C for 1 h with continuous agitation at 100 rpm (Fig. 1).
For SHF, 5 μL glucoamylase (Saczyme GO 2X, Novozymes, Bagsvaerd, Denmark) was added to the liquefied cassava solution, and the flask was incubated at 30°C and 150 rpm for 48 h until the glucose concentration increased. Concentrated nitrogen in a volume of 4 mL (100 g/L yeast extract and 200 g/L peptone) was added to 50 mL of saccharified cassava solution. Yeast cells were inoculated at an initial cell density of OD600=1.0. Fermentation was performed at 30°C and 150 rpm for 24 h until the ethanol concentration increased.
For SSF, 5 mL of glucoamylase, 5 mL of the nitrogen source, and 1.0 OD600, of the yeast cells were added to the liquefied cassava solution. Fermentation was performed at 30°C and 150 rpm for 48 h until the ethanol concentration increased. All fermentations were performed in triplicate. For comparison of fermentation parameters between SHF and SSF, Student’s t-test was performed at p<0.05.
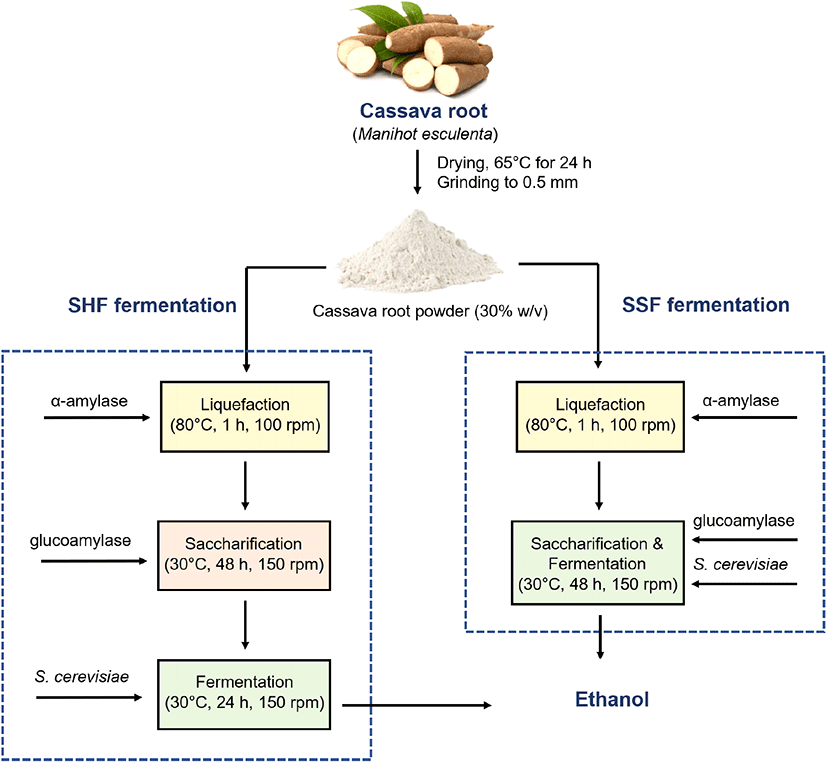
The glucose, fructose, glycerol, acetate, and ethanol concentrations of the hydrolysis and fermentation samples were analyzed through high-performance liquid chromatography (HPLC 1260 series, Agilent Technologies, Santa Clara, CA, USA) equipped with a Rezex-ROA Organic Acid H+ column (8%, 150 mm×4.6 mm; Phenomenex Inc., Torrance, CA, USA). The analytes were eluted with 0.005 N H2SO4 at 0.6 mL/min and 50°C, as previously described (Kim et al., 2019).
3. Results and discussion
To measure the total glucan content of cassava, α-amylase and glucoamylase were sequentially added according to the manufacturer’s recommendations, as described in the Materials and Methods. During glucoamylase treatment, glucose concentration was monitored every 12 h until it increased (Fig. 2). After α-amylase treatment with 30% (w/v) cassava powder solution (0 h), the concentrations of glucose and fructose were 33.0 and 5.6 g/L, respectively. When glucoamylase was added, glucose concentration significantly increased until 48 h, and the increase in fructose concentration was marginal. Lastly, from the saccharification of 30% (w/v) cassava powder solution, 254.1 g/L glucose and 14.7 g/L fructose were produced (Table 1). Using a conversion factor (1.1 g glucose/g glucan), it was determined that the 30% (w/v) cassava powder solution contained 231 g/L glucan, which accounts for 85.6% glucan (g/g dry matter) of the cassava powder.
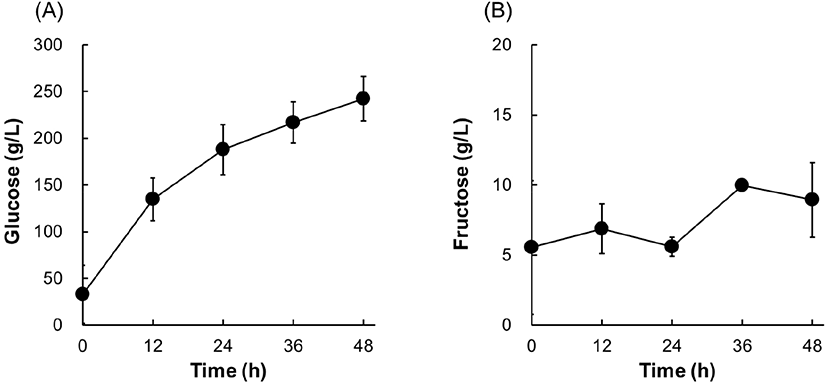
α-Amylase and glucoamylase are commonly used for the liquefaction and saccharification of starch (Yu et al., 1995). Sequential treatment of α-amylase and glucoamylase at their optimal temperatures (80-100°C and 30-50°C, respectively) efficiently yields glucose (Jadhav and Singhal, 2013). Fresh cassava contains 32-35% starch (80-90%, g/g dry matter) (Bantadjan et al., 2020), which is consistent with the results of this study.
For SHF, a saccharified solution of 30% (w/v) cassava powder was inoculated with S. cerevisiae EC1118, a commercial wine yeast strain. Fermentation was conducted at 30°C and the concentrations of ethanol and other metabolites were monitored every 12 h (Fig. 3). The glucose concentration decreased rapidly as the ethanol concentration increased linearly during 24 h of fermentation. At 24 h, glucose was depleted (256.5 g/L glucose consumed), and 130.6 g/L of the maximum ethanol concentration was achieved. Some fructose (11.9 g/L) was also consumed, and 9.5 g/L of glycerol was produced as a byproduct. Acetate concentration showed a slight reduction from 3.9 to 1.9 g/L. The ethanol yield was calculated as 0.49 (g ethanol/g sugar consumed), which was close to the theoretical maximum value (0.51 g/g). These results suggest that wine yeast EC1118 is suitable for cassava ethanol production at high solids loading (30%, w/v), resulting in >130 g/L ethanol at a near theoretical yield.
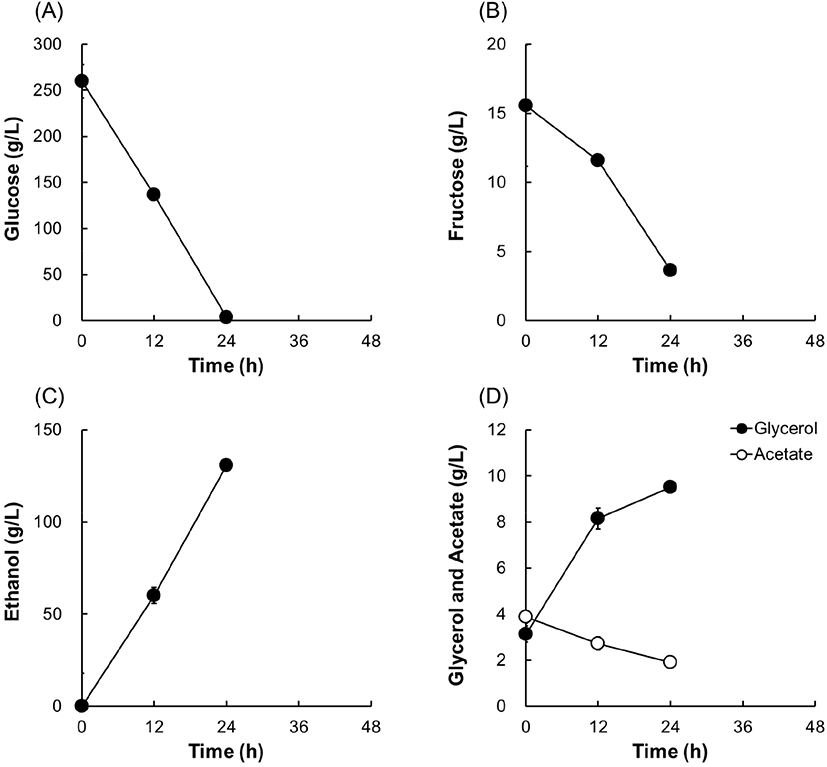
For SSF, the liquefied solution of 30% (w/v) cassava powder was mixed with glucoamylase and yeast cells, as described in Materials and Methods. During SSF at 30°C, the glucose concentration was maintained below 50 g/L and the ethanol concentration increased rapidly for 12 h (Fig. 4). Ethanol production continued for 48 h of fermentation, and the maximum ethanol concentration was 133.6 g/L. The final glycerol and acetate concentrations were 6.1 and 2.6 g/L, respectively. The ethanol yield was calculated as 0.51 (g ethanol/g sugar consumed). With respect to the maximum ethanol titer and yield, SSF had slightly higher values than SHF, although the difference was not statistically significant (Table 2). These results suggest that both SHF and SSF of high-solids cassava by wine yeast EC1118 can be used for efficient ethanol production.
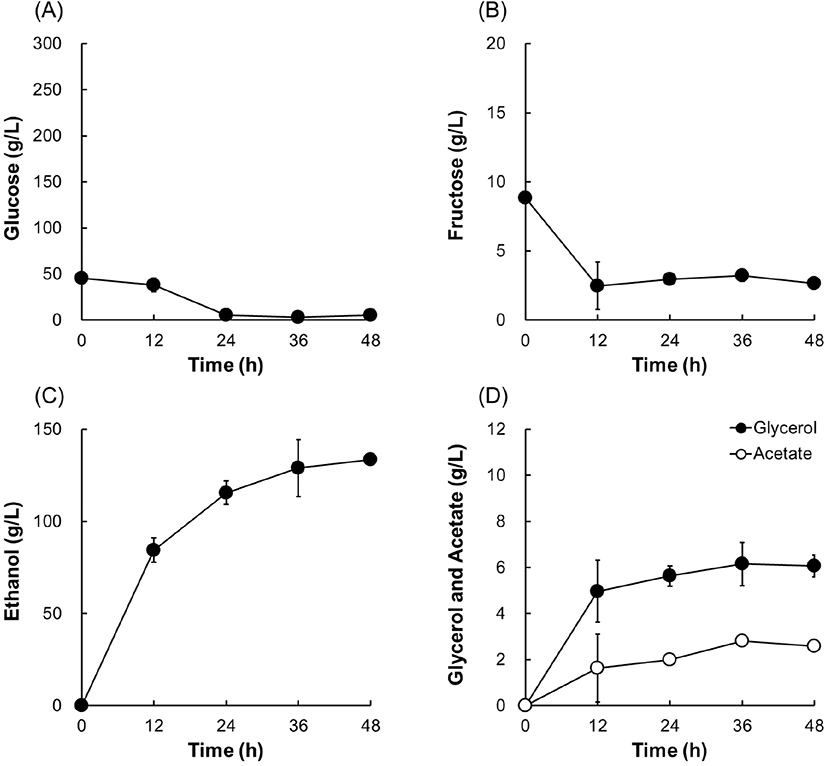
Fermentation mode | Cassava samples | Solid loading (w/v) | α-Amylase | Liquefaction conditions | Glucoamylase | Saccharification conditions | Fermentation conditions | Fermentation time | Ethanol (g/L) | Ethanol yield (g/g) | Reference |
---|---|---|---|---|---|---|---|---|---|---|---|
SHF1) | Starch | 20% | 0.9 mg/g | 85°C, 3 h | 1.5 mg/g | 85°C, 90 min | 10 L, 30°C, 70 rpm | 72 h | 43.5 | 0.44 | (Wangpor et al., 2017) |
Root | 35% | 150,000 U/mL | 93°C, 2 g, 2 h | 580 U/g | 61.5°C, 2 g, 48 h, pH 4.2 | 30 °C, 3 g | 48 h | 104.7 | 0.4 | (Sakdaronnarong et al., 2020) | |
Root | 30% | 0.33 mg/g | 80°C, 100 rpm, 1 h | 0.01% | 30°C, 150 rpm, 48 h | 30°C, 150 rpm | 24 h | 130.64 | 0.50 | This study | |
SSF2) | Pulp | 10% | 10.25 U/mL | 50°C, 2 h, pH 6.9 | 43.43 U/mL | 50°C, 2 h | 30 °C,100 rpm | 74 h | 27.4 | 0.29 | (Siriwong et al., 2019) |
Chip | 25% | 0.7 g/kg | 100°C, 90 min | 0.5 mg/kg | Continuous, 33 °C, 0.05 vvm | 24 h | 78.8 | 0.43 | (Choi et al., 2010) | ||
Starch | 20% | 0.3%, v/w | 40°C, 72 h | 0.1%, v/w | 60°C, 1 h | 5 L, 200 rpm | 72 h | 81.86 | 0.43 | (Krajang et al., 2021) | |
Root | 30% | 0.33 mg/g | 80°C, 100 rpm, 1 h | 0.01% | 30°C, 150 rpm | 48 h | 133.6 | 0.51 | This study |
The initial glucose concentration is one of the various factors affecting ethanol productivity. High concentrations of glucose can cause osmotic stress in yeast cells, especially when the initial cell density is low. In addition, osmotic stress can be one of the factors inhibiting the fermentation of high solids loading. Because hydrolyzed glucose is instantly consumed by yeast cells during SSF, the glucose concentration during fermentation can be kept low to a level that does not inhibit fermentation (Dahnum et al., 2015; Siriwong et al., 2019; Sovorawet and Kongkiattikajorn, 2012). In the present study, SSF showed significantly higher ethanol productivity (7.04 g/L-h) than SHF (5.01 g/L-h) during the first 12 h of fermentation. After 48 h of fermentation with sufficient cell growth, the ethanol productivity was not statistically significant (Table 1). The increased accumulation of glycerol in SHF might suggest that SHF was subjected to higher osmotic stress than SSF.
4. Conclusions
In the present study, it was confirmed that the commercial wine yeast S. cerevisiae EC1118 can produce >130 g/L ethanol from 30% (w/v) solid loading of cassava powder at a near theoretical yield. Both SHF and SSF have been successfully applied to cassava ethanol production. Although there was no statistically significant difference in ethanol titer and yield between SHF and SSF, SSF showed higher initial ethanol productivity and lower glycerol accumulation, suggesting less osmotic stress during the fermentation of high solids loading. The maximum ethanol concentration in this study was 133.6 g/L, which was the highest titer reported for cassava fermentation.