1. Introduction
Porridges are breakfast foods commonly eaten with soup or beans cake, and are also used as weaning food for babies and meals for patients, especially in developing countries in Asia and Africa, such as Nigeria and Kenya (Afolabi et al., 2018). It is commonly made from grains such as oats, maize, or sorghum, porridge. However, traditional porridge recipes based on cereals alone often lack essential nutrients, including protein, fibre, and vitamins, which are vital for maintaining good health (Sandhu et al., 2017). This nutritional gap limits the ability of porridge to meet daily dietary needs, making it important to explore ways to enhance its nutritional value (Ronto et al., 2018).
As awareness of health and diet increases, there is a growing demand for nutrient-dense and easy-to-prepare foods. Breakfast porridges could meet this demand. One promising approach to improving their nutritional profile is the use of composite flours, which are blends of starchy materials like cassava mixed with nutrient-rich ingredients. These composite flour blends can enhance the protein, fiber, vitamins, and antioxidants in porridge, making it a more nutritious meal (Emmanuel et al., 2019; Godswill, 2019).
Cassava, a widely consumed root crop in tropical regions, is rich in carbohydrates but has low protein and micronutrient content (Bayata, 2019). By incorporating other nutritious ingredients such as desiccated coconut and cocoa powder, the nutritional value of porridge can be significantly increased. Desiccated coconut is high in healthy fats, fiber, and minerals like potassium, magnesium, and iron, while cocoa powder is a rich source of antioxidants, especially flavonoids, which offer numerous health benefits (Glacometti et al., 2015). Combining these ingredients creates a balanced, health-promoting porridge that provides essential nutrients, energy, and compounds that support overall well-being.
Using composite flour made from cassava, desiccated coconut, and cocoa powder in porridge offers several advantages. The blend improves the porridge’s functional properties, such as water and oil absorption and gel formation, which contribute to a smooth, appealing texture (Osen et al., 2018; Malomo et al., 2012). Additionally, desiccated coconut and cocoa powder enhance the flavor and texture while boosting the antioxidant content, potentially reducing inflammation and stress in the body (Kourouma et al., 2019; Odunlade et al., 2016). Moreover, these ingredients provide a more complete and nutritious meal, supporting immunity, digestion, and heart health (Adeoye et al., 2020).
Although the benefits of composite flours are clear, there is limited research on the optimal ratio of desiccated coconut, fermented cassava mash, and cocoa powder in porridge. Most studies have focused on individual components or other flour blends, excluding fermented cassava or these particular ingredients. More research need to understand the nutritional, functional, and antioxidant properties and offer insights for creating healthier breakfast porridges. This study aims to explore the composition, functional characteristics, and antioxidant effects of a composite flour made from fermented cassava mash, desiccated coconut, and cocoa powder. It seeks to evaluate how this blend can be used to create a nutritious and functional breakfast porridge. By enhancing the nutritional and functional qualities of porridge, this research will offer a healthier alternative to traditional breakfasts and address nutritional deficiencies, particularly in areas with high rates of malnutrition.
2. Materials and methods
The farm at Obafemi Awolowo University Teaching and Research in Ile-Ife provided the fresh sweet variety cassava tubers (Manihot palmata). The coconut fruit was bought from Ile-Ife, Osun State’s central market. Cocoa Products (Ile-Oluji) Limited in Ondo State provided the cocoa powder.
The fermented cassava mash in Fig. 1 was made using conventional techniques as detailed by Obadina et al. (2012) and Akinwotu et al. (2020). Peeling and grinding the cassava roots into a pulp was the first stage. The pulp was stored in a bag and allowed to undergo fermentation for 72 h at room temperature. A local hydraulic press was used to crush the mash for 1 h after fermentation in order to eliminate extra water. After being hand mashed, the mash was sieved through raffia. It was sealed and placed into a hessian bag. The preparation’s cocoa powder was bought from Oluji, a business in Ondo State that is renowned for making premium cocoa powder.
As seen in Fig. 2, the desiccated coconut was made using the method described by Akinwotu et al. (2020). Initially, the dehusked coconut nuts were broken in half by hand hammering. A sharp knife was used to shell and peel the halves. Moulinex miller (Marlex Appliances Pvt. Ltd., Daman, India) was used to grind the coconut flesh into smaller pieces, but not to a silky texture. After that, the pieces were dried for 6 h at 80°C in a hot air oven. The coconut was dried, then mashed and kept at room temperature in a plastic container.
As indicated in Table 1, the initial phase of the study comprised weighing, separating, and combining the sample ingredients of water, desiccated coconut, fermented cassava mash, and cocoa powder in the following ratios: 70:0:0:30, 68:0:2:30, 54:16:0:30, and 52:16:2:30.
Sample1) | Cassava mash (%) | Desiccated coconut (%) | Cocoa powder (%) | Water |
---|---|---|---|---|
CM | 70 | - | 30 | |
CMCF | 68 | - | 2 | 30 |
CMDF | 54 | 16 | 30 | |
CMDCF | 52 | 16 | 2 | 30 |
CF | - | - | 100 | - |
DCF | - | 100 | - | - |
Moisture, crude protein, crude fat, crude ash, crude fibre, and N-free extract levels were determined using AOAC (Association of Official Analytical Chemists, 2016) standard procedures. The moisture content was measured by drying a 5 g sample at 105°C until its weight was constant. Crude protein was determined using the Kjeldahl method by measuring nitrogen and multiplying it by 6.25. Crude fat was extracted using the Soxhlet method with petroleum ether. Ash content was measured by burning a 2 g sample at 550°C in a muffle furnace. Crude fiber was measured by treating the sample with acid and alkali, then drying and weighing it. N-free extract content was calculated by subtracting the measured components from 100%.
Standard techniques were used to measure the functional characteristics of the composite flour blends, such as water absorption capacity (WAC), swelling capacity (SC), oil absorption capacity (OAC), and least gelation capacity (LGC), with some modifications made to account for the unique composition of the composite flour.
The American Association of Cereal Chemists’ (AACC, 1995) methodology was used to measure WAC. 1 g portion of the flour blend was placed in a graduated cylinder, 10 mL of distilled water was added, and the mixture stood at room temperature for 30 min. The volume that the sample took up was noted. WAC was computed as the volume growth as a percentage of the sample’s initial volume.
With a few adjustments, the Raikos et al. (2014) approach was used to calculate swelling capacity. A 25 mL graduated cylinder containing 0.5 g of the flour blend was heated for 30 minutes at room temperature, 60°C, 70°C, 80°C, and 90°C in a water bath. The sample’s final volume was noted once it had cooled. The percentage increase in volume following heating relative to the sample’s original volume was used to compute the SC.
The technique described by Osen et al. (2018) was used to test the flour mixes’ OAC. In a centrifuge tube, 10 mL of edible oil (sunflower oil) was added to a 1 g sample of each flour blend. After ten minutes of vigorous shaking, the mixture was allowed to stand for thirty minutes. The excess oil was decanted, and the volume of oil remaining in the flour was measured to determine how much oil had been absorbed by it. The outcome was given as a percentage of the oil’s initial volume.
The technique outlined by Sathe and Salunkhe (1981) was used to calculate the LGC, with modifications made by Godswill (2019). Two grammes of the sample were dissolved in one hundred millilitres of distilled water to create a 2% (w/v) solution of the flour blend up to 20%. After 30 min of heating at 90°C in a water bath, the mixture was allowed to cool to room temperature. The lowest concentration at which the gel formed and could stay erect without flowing was used to identify the LGC. The LGC was defined as the lowest concentration that resulted in a stable gel.
The antioxidant capacity of the flour blends evaluated are DPPH (2,2-diphenyl-1-picrylhydrazyl) radical scavenging activity and Total Phenolic Content (TPC).
The method used to measure the DPPH radical scavenging activity was based on Singleton et al. (1999) and Kourouma et al. (2019). A solution of 0.1 mM DPPH in methanol was made. Three millilitres of the DPPH solution were combined with one millilitre of the extract from each flour mixture. After shaking, the mixture was allowed to sit at room temperature for 30 minutes in the dark. A spectrophotometer was used to measure the absorbance at 517 nm. Using a particular formula, the radical scavenging activity was determined as the percentage of inhibition.
where Acontrol is the absorbance of the DPPH solution without the sample and Asample is the absorbance of the DPPH solution with the sample.
The Folin-Ciocalteu method, as described by Singleton et al. (1999) and Kourouma et al. (2019), was used to assess the total phenolics content (TPC) of the composite flour blends. One millilitre of the extract, one millilitre of Folin-Ciocalteu reagent, and two millilitres of a 20% sodium carbonate solution were combined. For thirty minutes, the mixture was left to stand in the dark. A spectrophotometer was used to measure the absorbance at 765 nm. By comparing the absorbance to a gallic acid standard curve, the TPC was determined in milligrammes of gallic acid equivalent per gramme of sample (mg GAE/g).
All experiments were performed in triplicate and represented as mean±SD (n=3). Data were analysed using the Statistical Package for the Social Sciences software (version 16.0; SPSS, Chicago, IL, USA). Significantly different treatments were detected by one-way ANOVA, and treatment means showing significant variation were further separated using Duncan’s multiple range test.
3. Results and discussion
The proximate composition of the composite flour blends is shown in Table 2, with moisture content ranging from 31.11% to 35.21%. Significant variations (p<0.05) in moisture content were observed across the samples. The raw cassava mash had a moisture content of 31.11%, while the enriched blends exhibited higher moisture levels. Cassava starch is known for its high water-absorbing properties, which contributed to the moisture in the blends. Despite the moisture-retaining nature of cassava starch, the moisture content of the cassava mash falls below the recommended 40-45% range for wet-based moisture (Kolawole et al., 2011). This suggests that while cassava starch influences the moisture content, it may also impact the storage properties of the composite flour. When 2% cocoa powder was added (Sample CMCF), the moisture level increased slightly to 31.80%, as cocoa powder contributes minimal moisture. In contrast, Sample CMDF, which contained 16% desiccated coconut, showed a higher moisture content of 34.43%, due to the natural moisture-absorbing ability of coconut. The highest moisture content (35.21%) was found in Sample CMDCF, which combined both cocoa powder and desiccated coconut. On the other hand, 100% desiccated coconut (Sample DCF) had moderate moisture at 6.35%, while 100% cocoa powder (Sample CF) exhibited low moisture at 5.28%, which is typical of dried ingredients. Both cocoa powder and desiccated coconut had moisture contents below the critical 10% threshold for proper flour storage (Assawarachan, 2021). These variations in moisture content are influenced by the water retention properties of each ingredient (Yakassai and Iguniwei, 2023). This study’s results are similar to Shakhawat et al. (2016), who found that wheat-coconut flour blends have increased moisture. This could be because coconut flour has a lot of fiber and fat, which helps it absorb moisture. This highlights that different ingredients have distinct effects on moisture retention in composite flour blends.
With values ranging from 3.22% to 7.44%, the protein content of the enriched cassava mash mixes also increased noticeably. Significant variations (p<0.05) in protein content were observed across the samples. Both Sample CM (2.51%) and Sample CMCF (3.22%), which were mainly made of cassava, had the lowest protein contents. Sample CMDF’s protein content increased by the addition of cocoa powder. Since coconut has a moderate protein concentration, samples CMDF (6.76%) and CMDCF (7.44%) that contained desiccated coconut had higher protein levels (Okafor and Ugwu, 2014). Given that cocoa is a strong source of plant-based protein, Sample CF (24.22%), which was made entirely of cocoa powder, had a much higher protein level. The protein content of desiccated coconut in Sample DCF (10.43%) was noteworthy, albeit lower than that of cocoa powder. The high protein level of both desiccated coconut and cocoa powder is primarily responsible for the increase in protein content. Although the protein level was less affected by the relatively small amount of cocoa powder (2%) added, the total increase in protein in the enriched blends was substantial (p<0.05). This is consistent with research by Bhagwat et al. (2014), which showed that including protein-rich ingredients increases the protein content of foods.
Additionally, the fat level of the enriched cassava mash mixes increased dramatically, ranging from 0.26% to 8.68%. Samples CM (0.20%) and CMCF (0.26%), which were made from cassava, had the lowest fat concentrations. Significant variations (p<0.05) in fat content were observed across the samples. Sample CMCF’s fat content marginally increased by the addition of cocoa powder. However, because dried coconut, which is known to have a high fat level, was added to Samples CMDF (8.21%) and CMDCF (8.68%), the fat content of these samples was higher. Due to the high fat content of cocoa, Sample CF (17.47%), which was made entirely of cocoa powder, had a higher fat level. Sample DCF, which was composed completely of desiccated coconut, had the highest fat level (28.90%). Medium-chain triglycerides (MCTs), which are advantageous for metabolism and energy production, are especially abundant in desiccated coconut (Trinidad et al., 2006). The 2% cocoa powder addition had little effect on the fat content, thus contributing less. These results are in line with earlier research that found comparable increases in fat content in mixes of cassava and tigernut flour, such as Sekinat et al. (2015).
The level of ash in cassava mash increased from 2.36% to 3.73% when cocoa powder and desiccated coconut were added. The sample including cassava mash and 2% cocoa powder had the lowest ash percentage (2.36%), while the sample containing cassava mash and 16% desiccated coconut had the highest ash content (3.73%). The ash level of these enhanced samples was significantly higher than that of the ordinary cassava mash (p<0.05). According to earlier research, this increase is probably caused by the higher ash content of desiccated coconut (7.15%) and cocoa powder (5.11%) (Okafor and Ugwu, 2014; Sekinat et al., 2015). The greater quantity of coconut utilised may potentially be the cause of the sample’s increased ash content (16% desiccated coconut). These findings demonstrate the nutritional advantages of including desiccated coconut and cocoa powder into cassava-based products, which may increase their mineral content and aid in the treatment of micronutrient deficiencies (Odunlade et al., 2016).
The fibre content of the enriched blends increased from 2.10% to 8.68% with the addition of desiccated coconut and cocoa powder. Significant variations (p<0.05) in fibre content were observed across the samples. Desiccated coconut had a greater impact on fibre levels, as seen by the similar fibre content of the sample with 16% desiccated coconut (Sample CMDF) and the sample with both 2% cocoa powder and 16% desiccated coconut (Sample CMDCF). The high fibre content of both cocoa powder and desiccated coconut is responsible for the increase in fibre content when compared to the plain cassava mash. Cocoa powder aids digestion and encourages fullness; in this study, it includes around 10.25% fibre (Sekinat et al., 2015). With 15.27% fibre, desiccated coconut is high in insoluble fibre, which lowers cholesterol, controls blood sugar, and promotes digestive health (Okafor and Ugwu, 2014). The notable increase in fibre content could be because of the combination of these components with cassava mash. This result is consistent with research by Sekinat et al. (2015), which indicates that incorporating desiccated coconut and cocoa powder into cassava increases fibre consumption and improves general health.
The N-free extract of the enhanced blends varied from 44.92% to 61.46%, with the plain cassava mash having the highest N-free extract content at 63.31%. Due to the high starch content of cassava, Sample CM had the most significant N-free extract content (63.31%). Due to the addition of cocoa powder, which is low in N-free extract, Sample CMCF (61.46%) had a somewhat reduced N-free extract content. Although, there was significant variations (p<0.05) in N-free extract content were observed across the samples. Even lower N-free extract contents were found in sample CMDF (46.43%) and CMDF (44.92%), since coconut has fewer carbs than cassava. The low N-free extract content of cocoa resulted in lower N-free extract levels in Sample CF (38.43%), which was composed entirely of cocoa powder. Sample DCF, which was composed completely of desiccated coconut contains lower N-free extract than cassava, had the lowest N-free extract level (32.75%). The N-free extract content of the enhanced blends was decreased (p<0.05) by the addition of desiccated coconut and cocoa powder. This decrease is because part of the N-free extract were replaced by increased amounts of ash, protein, fat, and fibre. Cocoa powder, which adds protein and fibre, and desiccated coconut, which is high in fat and fibre, helped improve the nutritional profile while reducing the N-free extract level. These findings are consistent with research by Ajibola et al. (2015) and Bolarinwa et al. (2017), who also found that adding ingredients high in protein, fat, and fibre to food formulations reduced the amount of carbohydrates. Although N-extract of the blends were determined in this study.
WAC, SC, OAC, and LGC are among the functional characteristics of cassava-based mixes that are displayed in Table 3. At room temperature, the mixes’ WACs varied from 32% to 51%, with the enriched mixes showing the highest variances (p<0.05). In particular, among the enriched samples, the mixtures with desiccated coconut had the lowest WAC, at 32%. The high fat content of desiccated coconut (17.47%) may be the cause of this reduced WAC since it forms a hydrophobic barrier that hinders the flour matrix’s ability to absorb water (Godwill, 2019). When compared to other samples, the WAC is lower because the fat in desiccated coconut repels water (Siddiq et al., 2015). Conversely, the mixtures with cocoa powder exhibited a slight increase in WAC. Significant variations (p<0.05) in WAC were observed across the samples. Due to its amorphous nature and the presence of hydrophilic groups, including hydroxyl groups, which form hydrogen bonds with water molecules, cocoa powder has a higher potential to absorb water (Galet et al., 2004). These interactions allow cocoa powder to bind water more efficiently, as seen by its greater WAC (132%) (Kakar et al., 2022). The higher WAC in combinations enhanced with cocoa powder because of this characteristic. The results of earlier research are consistent with the lower WAC in mixes enriched with desiccated coconut and the higher WAC in mixes loaded with cocoa powder. When cocoa powder was added to sorghum ogi, for example, Odunlade et al. (2016) observed a comparable rise in WAC, which they attributed to the powder’s capacity to bind water. In foods like soups, bread, and baked goods, water absorption plays a crucial role.
The mixtures’ OACs varied from 21% to 33.35%. The mixture including desiccated coconut had the lowest OAC, most likely because of its low WAC and high fat content, which limit its capacity to absorb more oil. Significant variations (p<0.05) in OAC were observed across the samples. The high fat content (17.47%) of desiccated coconut limits its capacity to absorb oil by making the surroundings more hydrophobic. This is consistent with the well-known idea that food matrices with a high fat content might decrease the absorption of other liquids, such as oil (Godwill et al., 2019). Additionally, as these two properties are commonly inversely related, the reduced WAC in the desiccated coconut-containing mixes could be a factor in their lower OAC (Mandilik et al., 2018). Despite this, the mixture with cocoa powder experienced a moderate OAC increase of 33.35%. Due to the presence of protein functional groups, cocoa powder, which has a high protein content (around 24.22%), might enhance the absorption of fat (Galet et al., 2004). Proteins interact with oil to increase its absorption capacity, especially those that contain hydrophobic amino acids (Kakar et al., 2022). Furthermore, the protein in cocoa powder gives oil molecules additional binding sites, which promotes absorption. Higher OAC enhances flavour retention and produces a smoother texture in foods high in fat, making it a beneficial feature in food composition (Shreshtha and Srivastava, 2017). The potential application of cocoa powder-enriched mixtures in food products where oil integration is crucial is enhanced by their higher OAC. Because it helps the product retain oils, preventing rancidity and preserving freshness, this can result in enhanced flavour retention, an improved mouthfeel, and potentially a longer shelf life (Suresh and Samsher, 2013).
The blends’ LGCs varied from 5% to 7%. With the lowest LGC of 5%, Sample CM, which only included cassava mash showed good gelling capacity. The starch included in cassava mash is essential for gel production because it can absorb water and solidify into a gel when heated. Cassava mash’s low LGC suggests that it gels readily at lower concentrations, which is characteristic of starches with a high amylopectin content (Ammar et al., 2016). As a result, cassava mash is a useful gelling ingredient in food compositions, particularly in porridge and other dishes that are gel-based. The distinct ingredients’ gelling qualities are responsible for the variations in LGC seen in the enriched mixes. The final mix’s gelling ability may be impacted by the interactions between the lipids, proteins, and carbohydrates in the desiccated coconut and cocoa powder. Due to its high fat content, desiccated coconut may decrease the absorption of water, hence reducing the production of starch gel. Through interactions between proteins and starch, cocoa powder, which contains certain proteins and fibres, may also have an impact on gelling (Noorfarahzilah et al., 2014). These components change the mix’s molecular interactions and water-binding qualities, which affects gel formation (Chandra and Samsher, 2013). Although the LGC of the enhanced mixes containing desiccated coconut and cocoa powder did not change much, the higher carbohydrate content of cassava mash resulted in a stronger gelling ability. The concentration of starch, the main carbohydrate in cassava, has a direct effect on the LGC and is essential for gelation (Mandilik et al., 2018). In comparison to pure cassava mash, the addition of fat and protein-rich ingredients such as desiccated coconut and cocoa powder probably diluted the starch content, resulting in a greater LGC and a lower gelling ability (Raikos et al., 2014).
The SC of the mixtures is displayed in Fig. 3 and ranges from 255% to 377% at 90°C to 39% to 65% at ambient temperature. Room temperature had the lowest SC and 90°C the highest. In starch-based mixes, this temperature-dependent increase in SC is common because starch granules swell more at higher temperatures because of increased water absorption and gelatinisation (Godwill, 2019). Higher SC values result from the expansion, breakdown, and absorption of water by starch granules caused by heat (Raikos et al., 2014). Since starch granules do not swell much in the absence of heat, the SC was lower at room temperature, which is consistent with research that indicates SC rises with temperature (Li and Gilbert, 2018). Cocoa powder and desiccated coconut had a major impact on the SC of the enhanced mixes. Relatively low SC values ranged from 19% to 39% for mixtures including desiccated coconut. This is due to the high fat content of desiccated coconut, which coats starch granules and inhibits their ability to properly absorb water and swell. This finding is in line with research by Singh et al. (2003) and Miao et al. (2018), which demonstrated that a high fat content could prevent starch swelling by reducing the starch-water interaction. These results of functional properties of the blends show how desiccated coconut and cocoa powder alter the functional characteristics of cassava, enabling it to be used in a variety of culinary applications.
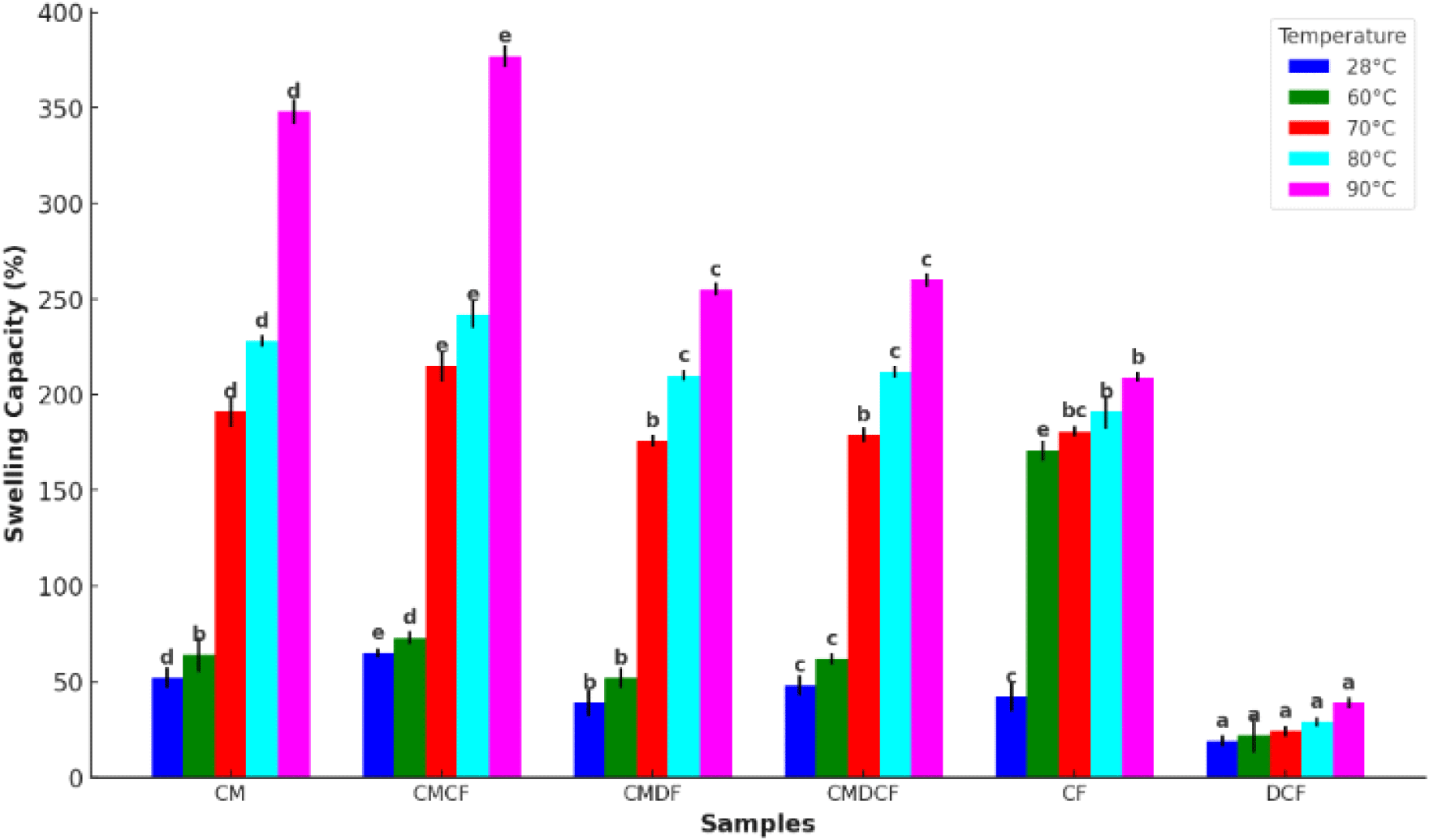
The mathematical relationships for swelling capacities at room temperature, 70°C, 80°C, and 90°C are shown in Table 4. In order to explain the swelling behaviour of cassava mash, cocoa powder, and desiccated coconut powder at these temperatures and show how temperature variations affect the blends’ swelling capacity, the table provides regression models that use linear, logarithmic, polynomial, and power equations. For polynomial (R2), linear (R12), exponential (R22), logarithmic (R32), and power law (R42) equations, the models are assessed according to their respective R2 values.
A significant correlation between swelling capacity and the amount of cocoa powder in the mix was indicated by the R2 values for the linear model, which ranged from 0.6878 to 0.9228, with 100% cocoa powder showing the highest value of 0.9228. With rising values closely matching the swelling capacity as a function of temperature and constituent mix, the linear model demonstrated a satisfactory fit. The coefficients for the logarithmic model varied from 0.5465 to 0.982, with cocoa powder once more receiving the highest value. According to this, the swelling capacity of cocoa powder blends increased quickly at first and then more gradually as the temperature rose, exhibiting a logarithmic pattern with regard to temperature.
According to the logarithmic model’s strong fit, mixes with larger amounts of cocoa powder exhibit this growth pattern more prominently. With R2 values ranging from 0.8001 to 0.8625 for the exponential model and from 0.677 to 0.9495 for the power law model, the two models likewise demonstrated strong correlations. These findings imply that the swelling capacity of mixtures grows more rapidly with increasing temperature, particularly for mixes that contain additional constituents (Hussain and Uddin, 2012). The power law model, which links ingredient composition, temperature, and swelling capacity, aids in explaining the overall pattern, while the exponential model demonstrates that the swelling rate increases more quickly at higher temperatures.
With R2 values ranging from 0.9171 to 0.9916, the polynomial equation was the model that best suited the data. This shows that the polynomial model best represents the intricate interaction between temperature, components, and swelling capacity. The polynomial model effectively explained the majority of the variation in swelling capacity at various temperatures, as seen by the R2 values being near 1. The polynomial equation was the most accurate model, but all of the models fit the data well and had no discernible problems. These results are consistent with Singh and Pandey’s (2011) research, which demonstrated how effective polynomial equations are in properly simulating intricate food characteristics like swelling capacity. This work illustrates how mathematical models can be used to comprehend the effects of components, temperature and swellling capacity of the cassava-based mixtures.
The impact of enrichment on the cassava-based mixes’ TPC and DPPH radical scavenging activity is displayed in Table 5. The unenhanced cassava mash (CM) had a DPPH scavenging ability of 45.0%, while the other combinations’ ranged from 41.82% to 62.02%. When compared to the unenhanced cassava mash, the enriched mixes with cocoa powder (Samples CMCF and CMDCF) demonstrated a substantial improvement (p<0.05) in DPPH scavenging ability. The high polyphenol content of cocoa powder (76.47%), which is well-known for its potent antioxidant qualities, is probably the cause of the enhancement in radical scavenging ability in the cocoa-enriched mixes (Glacometti et al., 2015). It is well known that polyphenols, particularly the flavonoids in cocoa, have potent anti-free radical properties, which may account for an increase in DPPH scavenging observed presently in the study. These outcomes are consistent with those of Odunlade et al. (2016), who found that adding cocoa powder to sorghum ogi improved DPPH scavenging in a comparable way. Urbanska et al. (2019) also emphasised how cocoa might boost food’s antioxidant qualities and counteract free radicals. The addition of cocoa powder to cassava mash appears to assist the mixture combat oxidative stress by neutralising dangerous free radicals, which is crucial for maintaining health, as seen by the increase in DPPH scavenging ability. This study demonstrates how cocoa powder can increase food items’ antioxidant content, providing further health advantages, particularly in the production of functional foods. However, the variable quantities of raw components with different phenolic contents affected the DPPH scavenging performance.
The TPC level of the mixes, which ranged from 15.54 mg GAE/g to 16.84 mg GAE/g, was considerably higher than that of cassava mash alone, which had a total phenolic value of 10.61 mg GAE/g. The total phenolic content increased significantly by the addition of cocoa powder, and the values of the mixes including cocoa powder were significantly greater than unenriched ones (p<0.05). This rise is consistent with the results of Odunlade et al. (2016), who discovered a comparable pattern in sorghum ogi, wherein the total phenolic content increased with the addition of more cocoa powder. This study’s higher phenolic content (20.12 mg GAE/g) is probably due to the high concentration of polyphenols in cocoa powder, which is known to have strong antioxidant properties (Ahmed et al., 2020). Of all the blends, the blend containing both desiccated coconut and cocoa powder had the highest phenolic content, probably because the two ingredients combined to increase the overall phenolic levels. The blend containing only 100% cassava mash had the lowest phenolic content, given its low polyphenol content. While desiccated coconut is not as rich in phenolics as cocoa powder, it nevertheless adds extra nutrients that can boost the antioxidant potential when mixed with cocoa powder. This implies that adding desiccated coconut and cocoa powder to cassava mash results in a more potent supply of phenolic compounds, increasing its nutritional value and maybe providing health advantages due to its enhanced antioxidant qualities.
4. Conclusions
The nutritional, functional, and antioxidant properties of cassava mash enriched with desiccated coconut and cocoa powder were examined in this study. The results demonstrated that the addition of desiccated coconut and cocoa powder greatly enhanced the nutritional makeup of cassava mash by raising its levels of phenolics content, protein, fat, and fibre. Significant improvements were also made to the functional properties of enriched mixture, which made them more appropriate for a variety of food processing applications. These improved qualities included swelling capacity, oil absorption capacity, water absorption capacity, and gelation characteristics. The mixture of 16% desiccated coconut and 2% cocoa powder yielded the best results out of all the combinations tested; it increased the antioxidant activity of the cassava mash and improved its nutritional value and functional qualities. This mixture offers the potential to provide a healthy and nutritious porridge substitute. These advancements suggest that composite flours containing desiccated coconut, cocoa powder, and cassava mash may be valuable for food manufacturing, especially for functional foods like breakfast porridges. These enriched blends offer a more balanced, antioxidant-rich, and fiber-rich food product that promotes general health and well-being, which may help close nutritional gaps in various populations.