Introduction
Recently, in order to increase sustainability in processing industries, focus has been placed on the exhaustive use of fruit and vegetable waste biomass, particularly from plant parts such as seeds, husks and roots. Among them, peanut hulls have shown considerable applications in multiple industries and a number of ventures have been undertaken in order to increase their use (Zhao et al., 2012). Peanuts (Arachis hypogaea L.) are a major international cash crop as the kernel is used for a variety of products, such as snacks, peanut butter and oil (Lee et al., 2011). The skins and hulls, however, are often taken as agricultural waste; used in compost, fuel, feed or simply abandoned (Qiu et al., 2018; Win et al., 2011).
Approximately 230-300 g of hulls are produced per kilogram of peanut and the increasing annual production gives rise to over 13.5 million metric tons of the shells (Zhao et al., 2012). Peanuts hulls are composed of cellulose (40.5%), lignin (26.4%), hemicelluloses (14.7%) trace macro and micro-nutrients, as well as phenolic compounds such as eriodictyol, 5,7-dihydroxychromone, and luteolin (Lee et al., 2008; Qiu et al., 2018; Rivilli et al., 2012). Luteolin is the most abundant phytochemical extract, chemicals from plant origins, of the phenolic compounds in peanuts hulls and it is a flavonoid with various pharmacological and antioxidant properties (Adhikari et al., 2018; Lee et al., 2008). Moreover, it has been shown to have high bioavailability when sourced from peanut hulls (Zhou et al., 2008). However, luteolin extraction is influenced by polyphenol-cell wall interactions that have been proposed to involve non-covalent associations, including hydrogen bonding, hydrophobic forces and ionic interactions, all of which can be affected by extraction conditions of temperature, time and pH (Boonmee, 2012; Phan et al., 2016). Therefore, it is necessary to break the bonds, by cleavage and cell wall disruption, for its release (Bohn, 2014).
Various methods, such as enzymatic hydrolysis (Chun et al., 2010), roasting (Taha et al., 2012), solid state fermentation (Liu et al., 2018) have been used to degrade the cellulose matrix of peanut shells. Efficient degradation has also been achieved through a combination of mechanical and thermo- alkaline pre-treatments (Dahunsi et al., 2017).
The purpose of this study was to determine the optimal conditions of temperature and extraction solvent concentrations for luteolin extraction from peanut hulls. In addition, the antioxidant component contents and antioxidant capacities of extracts were measured. The results of the study are intended to contribute to the extensive utilization of peanut hull waste by obtaining bioactive compounds prior to secondary processes such as biorefinery and composting.
Materials and methods
Peanuts were obtained from a local market in Gochang County, South Korea. Peanuts were washed and dried using a convection oven (FC-2D-2S, Universal Scientific Co., Shanghai, China) at 60°C until a moisture content of 7% was reached. They were then sorted and shelled. Dried shells were crushed into a powder, passed through a 40- mesh sieve and placed in storage at 4°C until analysed. Gallic acid, ascorbic acid, quercetin, 2,2-diphenyl-1- picrylhydrazyl (DPPH), 2,2'-azino-bis(3-ethylbenzothiazoline- 6-sulphonic acid) (ABTS), aluminium chloride hexahydrate and sodium carbonate were purchased from Sigma Chemical Co. (St. Louis, MO, USA). Folin-Ciocalteu reagent was acquired from Junsei Chemical Co. Ltd. (Tokyo, Japan). Luteolin was purchased from Wako Chemicals Inc. (Richmond, VA, USA) and HPLC-grade ethyl alcohol, acetonitrile, glacial acetic acid and water were purchased from Fisher Scientific Inc. (Fair Lawn, NJ, USA).
During preliminary testing, 3 h and 12 h extractions were performed to select a basis for an extraction time. One gram of peanut hull powder was extracted in 50 mL of solvent for 3 h under reflux extraction. For comparison, conventional stirring was also performed for 3 h.
To determine the best methanol concentration, peanut hull powder was extracted in methanol at concentrations of 20, 40, 60, 80 and 100%. The hull powder was also extracted in distilled water as the control. These mixtures were extracted under reflux at equidistant temperatures of 25, 55 and 85°C in a water bath. Following this, sample extracts were filtered (0.45 μm, polyvinylidene fluoride filters) and centrifuged at 10,000 ×g for 10 min at 4°C. Supernatants were collected for subsequent analyses.
The TPC of the crude methanolic extracts were analysed using the Folin-Ciocalteu method with some modifications. One milliliter of sample extract was mixed with 5 mL of Folin-Ciocalteu reagent and let to stand for 3 min. Four milliliters of 7.5% sodium carbonate was added and the mixture was developed in the dark before its absorbance was read. Gallic acid solution with a series of concentrations was used as the standards. The absorbance was measured at a wavelength of 765 nm with the UV-1700 PharmaSpec UV-visible spectrophotometer (Shimadzu Scientific, Kyoto, Japan). The TPC was expressed as mg of gallic acid equivalent per 100 g dry peanut hull powder.
The TFC of the peanut hull extracts was measured using the aluminium chloride colorimetric method (Kalita et al., 2013) with some modifications. One millilitre of sample extract was mixed with 150 μL of 5% sodium nitrate. This was left at room temperature for 5 min and 300 μL of aluminium chloride hexahydrate was then added. The mixture was then let to stand for 6 min. One millilitre of 1 M sodium hydroxide was added and mixed with 550 μL of water prior to reading the absorbance. Quercetin was used as the standard, prepared in 95% ethanol. Absorbance was measured at 510 nm and TFC was expressed as mg of quercetin equivalent per 100 g dry peanut hull powder.
Thirty microliters of sample extract was mixed with 3 mL of 2.45 mM prepared ABTS (having an absorbance of 0.7±0.02) and let to stand in the dark for 6 min. Absorbance was measured at a wavelength of 734 nm and the scavenging activity was determined according to the following formula:
Varying concentrations of L-ascorbic acid were used to produce a standard curve from which the IC50 value was determined, expressed as μg/100 mg vitamin C equivalent antioxidant capacity (VCEAC).
One hundred microliters of extract was mixed with 2 mL of 100 μM DPPH solution and left to develop in the dark for 20 min. Absorbance was measured at 515 nm and the scavenging activity was determined according to the formula:
Varying concentrations of L-ascorbic acid were used to produce a standard curve from which the IC50 value was determined, expressed as μg/100 mg VCEAC.
The analysis of luteolin standards and peanut extracts was carried out on a Waters Alliance e2695 Separations Module (Waters Co., MA, USA) using a Waters 2998 Photodiode array detector. The machine was equipped with Empower software (Europa Science Ltd., Cambridge, UK) and compounds were separated on a C18 reverse-phase column maintained at ambient temperature. The mobile phase had a constant flow rate of 0.80 mL/min and was used with two solvents (A and B). Solvent A was 0.1% formic acid in water (HPLC grade) and solvent B was 80% acetonitrile and 20% acetic acid. The elution conditions were as follows: 15-40% B for 30 min, 40-100% B from 30-40 min, and 100% B from 40-47 min. The injection volume was 20 μL and detection was at 263 nm.
Under response surface methodology, the Central Composite Design was used to determine the optimal conditions of the controlled parameters at which the extracts would exhibit high antioxidant potential. The two factors were applied at three levels (Table 1).
The quality of the model was fitted onto the quadratic model as:
where: Y = response variable; β0 = intercept; βi = first order coefficient of the model where i = 1, 2… k); βij = interaction effect; βii = quadratic coefficients of Xi and ɛ = random error.
The data collected for the responses (Y) were luteolin, TPC, TFC, ABTS and DPPH activity, and these were analysed via Stat-Ease Design-Expert software package (11.1.2.0, Stat-Ease Inc., Minneapolis, USA) and SigmaPlot (13, Systat Software Inc., California, USA). Mean separations were obtained using Tukey’s student test at p≤0.05.
Results and discussion
The recovery of polyphenolic compounds from plant tissue often employs the use of polar solvents and methanol has been shown to typically be efficient in the extraction of compounds with lower molecular weights (Do et al., 2014). In addition, higher amounts of polyphenols can be extracted with an increase in the polarity of the solvent. In addition, higher amounts of polyphenols can be extracted with an increase in the polarity of the solvent. A report on the various solvent extracts of phenolics from moringa leaves (Siddhuraju and Becker, 2003) stated that methanol was the most ideal solvent for phenolic extraction because of its high polarity and the phenolic compound solubility of plant components.
During preliminary testing, 3 and 12 h extractions were performed to select an extraction time and there were no increases in the luteolin content after 12 h. Extracts from conventional stirring after 3 h were 122.13 mg/100 g while 12 h extracts had 119.38 mg/100 g luteolin. Twelve-hour reflux extracts had a luteolin content of 173.46 mg/100 g and three-hour extracts had a content of 174.03 mg/100 g. For both extraction methods, 3 h was selected as the extraction time.
In this study, at 80% methanol concentration, luteolin, polyphenols and flavonoid content were found to be the highest (Fig. 1). This variation was likely due to the increased efficiency of the extraction of phenolic compounds and flavonoids which is influenced by the polarity of the compounds. To confirm this, conventional stirring was performed using 80% methanol and the antioxidant compound contents and capacities of the extracts were determined. The luteolin content, antioxidant contents and antioxidant capacities of reflux extracts generated at 85°C in 80% methanol were generally higher than that of conventional stirring. The luteolin contents of the extracts were 122.13 mg/100 g from conventional stirring and 174.03 mg/100g from reflux extraction. For the experimental means from the reflux extracts, the TPC was 2,049.51 mg GAE/100 g; TFC was 1,817.86 mg QE/100 g; ABTS scavenging activity was 67.21%, and DPPH scavenging activity was 81.10%. For conventionally stirred extracts, TPC was 844.75 mg GAE/100 g; TFC was 780.0 mg QE/100 g; ABTS radical-scavenging activity was 41.13%, and DPPH scavenging activity was 62.48%. The IC50 values for ABTS and DPPH radicals were 104.18 μg/mL and 83.08 μg/mL, respectively.
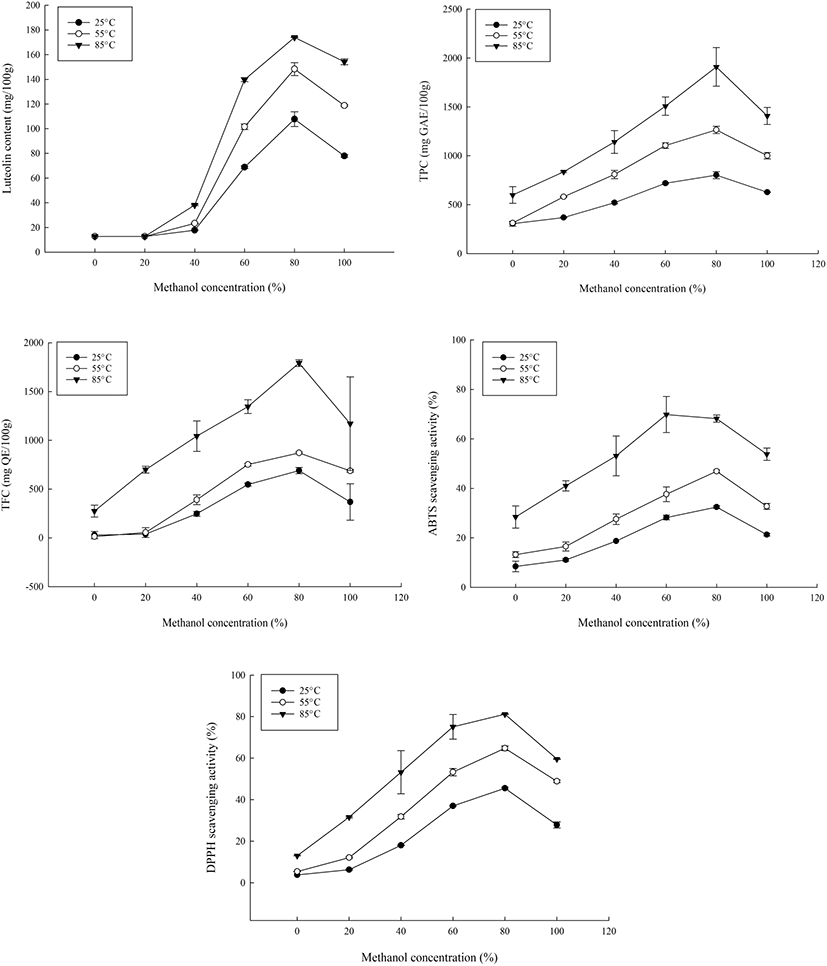
Extractions performed at room temperature had the lowest contents for all five parameters measured and raising the temperature led to an increase in the luteolin extracted as well as the antioxidant contents and capacities. Refluxing at room temperature (25°C) produced a luteolin content of 107.75 mg/100 g which was lower than that of stirring. However, the reflux extract at 85°C (174.03 mg/100 g) increased the luteolin content by 42.50%. Overall, higher temperatures led to higher luteolin and antioxidant contents, which was a different finding from vortex mixing which exhibited no improvements upon comparing room temperature and 50°C extractions (Lee et al., 2008).
The processes of heating and hydrolysis reactions are both able to increase the polyphenol content during extractions by releasing compounds that were previously bound to cell wall components. Heat in particular liberates and activates low molecular weight polymeric subunits (Hatam et al., 2013) which allows higher temperatures to be used in extractions without degrading the desired phenolic compounds. In addition to this, heating under reflux provides continual mixing of the solution, improving the extraction efficiency. The higher temperatures result in increased diffusion and improved solubility and the kinetic energy is distributed at a molecular level, which stirring alone may not achieve.
For all studied parameters, a concentration of 80% methanol and temperature of 85°C increased the phenolic compounds as well as their antioxidant capacity (Fig. 1). There were significant differences between the temperature and methanol concentrations of the extracts (p<0.05), with that of temperature being highly significant (p<0.01) due to enhanced extractions and recovery of phenolic compounds through the increase of the diffusion coefficient and solid to solvent solubility. However, prolonged exposure or treatment with high temperatures could also lead to deterioration of active compounds which is likely the cause of the eventual decrease.
At all temperatures, the highest yield of compounds was obtained from the 80% methanol extracts and while some compounds could be water-soluble, the efficiency of water as an extraction solvent, unaided by other factors like temperature, has been shown to be lacking in previous studies (Boonmee, 2012; Do et al., 2014; Kalita et al., 2013).
To determine the optimum extraction conditions of the peanut hulls, the central composite design was used (Table 1, Table 2). The lack of fit for the measured parameters was insignificant relative to the pure error and this was tested against each response. Non-significant lack-of-fit is the preferred state of the model and so, implied the model fit for the responses. For TPC, due to the wide range present between the experimental values, the data was transformed and analyzed. The results obtained showed that there was less efficient extraction occurring at lower temperatures and lower methanol concentrations for all responses (Table 3, Table 4). At 25°C, luteolin ranged from 68-112 mg/100 g while TPC, TFC, ABTS and DPPH ranges were 628.22- 828.22 mg GAE/100 g, 235.81-713.23 mg QE/100 g, 20.93- 32.08% and 28.92-45.88%, respectively. The observed tendency showed that 100% methanol had the least antioxidant activity and content while 80% methanol had the highest at that temperature. This was similarly the case at 85°C, where the response variables ranges were 152.57- 174.12 mg/100 g for luteolin content; 1,346.6-1,769.9 mg GAE/100 g for TPC; 832.14-1,767.86 mg QE/100 g for TFC; 52.06-75% ABTS scavenging activity, and 59.7-81.3% DPPH scavenging activity. These values were from lowest at 100% methanol to highest at 80% methanol, with the exception of ABTS scavenging activity which was highest at 60% methanol.
From the CCD, the predicted optimal conditions for maximum yield of luteolin, were: 81.22°C and 87.47% methanol for a predicted value of 174.51 mg/100 g (Table 5). These experimental conditions were applied as 81°C and 87.5% methanol, and to verify the accuracy of the predicted optimal parameters from the CCD (X1: 87.5% and X2: 81°C), the luteolin content was determined under these optimal conditions. The percentage difference between the values obtained by the model were all below 10% which confirmed the predictability of the model for the extractions of peanut hulls. To confirm optimization, extraction was performed at 90°C and the luteolin content decreased to 68.56 mg/100 g, suggesting possible degradation at that temperature.
The interaction effects of the factors on the compounds and activities was shown on surface plots (Fig. 2) that expressed the interactive relationships between the methanolic concentration and temperature of the different extracts. Higher temperatures can enable the degradation of the cell walls of the peanut hulls but also increase the degradation of flavonoids like luteolin, in addition to degrading thermal labile phenolic compounds. With the exception of the methanol concentration, there were significantly positive correlations between the response variables as well as between the response variables and increasing extraction temperature. The parameters all had significant correlations between them (R2>0.70). The antioxidant components of phenolic and flavonoid compounds were reflected in the linear correlations between the parameters of phenolics, flavonoids, ABTS scavenging activity and DPPH scavenging activity. The increased luteolin content and antioxidant potential of peanut hull extracts can enhance the stability of various products if applied against primary oxidation.
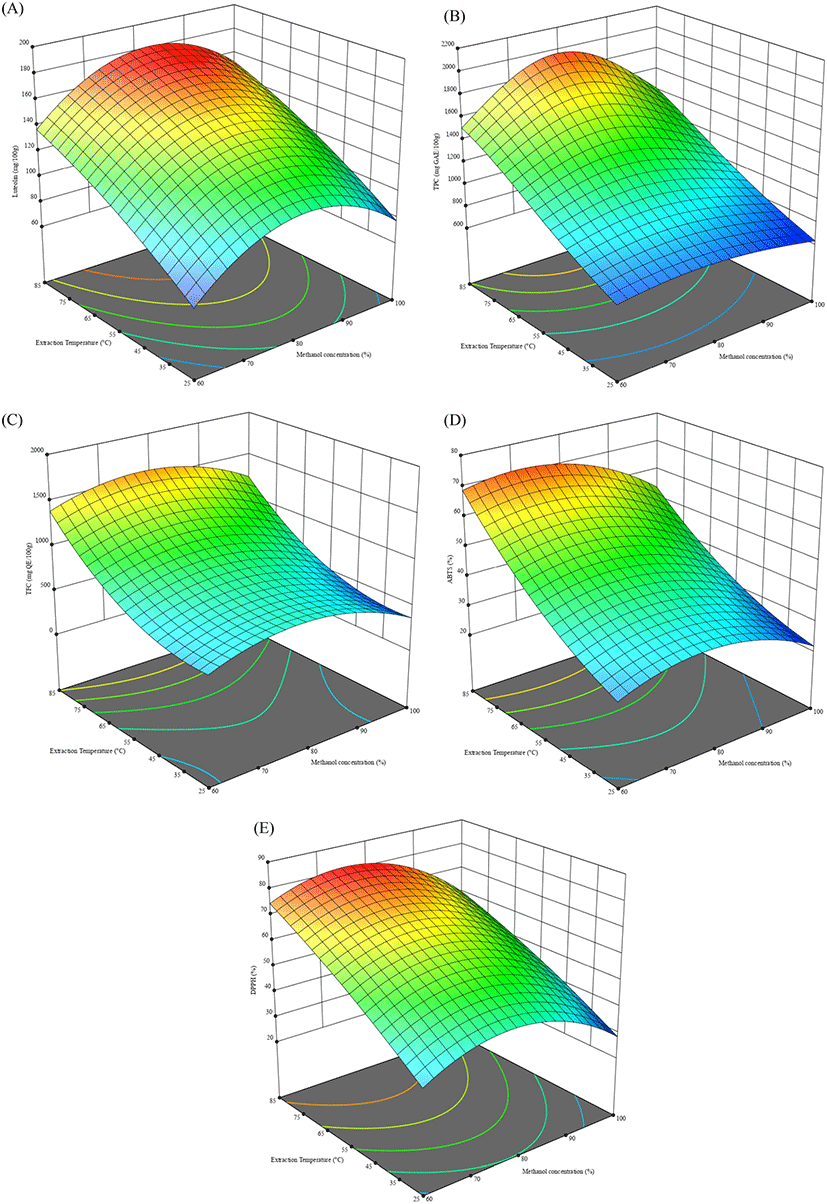
Conclusion
Extracting luteolin under reflux from peanut hulls is a simple method with relatively low costs that, in succession, can exhaustively utilize peanut waste biomass in the production chain. Temperature and methanolic concentrations were optimized for increased extraction efficiency with the added benefit of increasing the antioxidant content and activity of the extracts and the extracted luteolin was increased by 42.50%. The results of the study will contribute to the extensive utilization of peanut hull waste by obtaining bioactive compounds prior to secondary processes such as biorefinery or composting facilities.