Introduction
Tomato (Solanum lycopersicum Mill.) is an economically important crop in the world due to the demand for the fresh and processed vegetable food industry and its enrichment of vitamins and minerals (Klee and Giovannoni, 2011; Rai et al., 2013). In general, tomato varieties are commonly classified into two types according to their color; pink-colored tomato and red-colored tomato. In Asia, pink-colored tomatoes are very popular for consumption (Ballester et al., 2010); in particular, one of the pink-colored varieties-‘Dotaerang’-is widely grown in Korea. Pink-colored tomatoes have higher sugar content than red-colored tomatoes, but they have fruit cracking problems during cultivation and transportation due to their lower firmness. Both tomatoes are a climacteric fruit, and their ripening processes are accompanied by changes in physical, chemical, and physiological characteristics of fruit with effects from exogenous and endogenous ethylene production (Majidi et al., 2012). There is a rapid increase in ethylene production and the respiration rate during the ripening stage and also after the harvest period (Zhang et al., 2017).
Low temperature is an essential factor to extend the storage life and control ethylene production and respiration. Low temperature storage increases the time to ripening and shelf life and reduces the deterioration of product quality by controlling physicochemical and microbiological activities (Tano et al., 2007; Wang et al., 2008). However, tomatoes-like tropical and subtropical crops-are vulnerable to chilling injury (CI) when stored at low temperatures after harvest (Ding et al., 2002). In tomato, non-uniform ripening, pitting, water-soaked areas, and fungal infections have already been reported as economic losses due to CI (Liu et al., 2012). CI is a physiological disorder of a plant and plant organs caused by exposure to chilling temperature. For long-distance transportation in long-term cold chain system, CI must be overcome. In various fruits and vegetables, high CO2 levels maintain fruit quality and improve tolerance of fruit during low temperature storage (Besada et al., 2015; Dong et al., 2015; Vazquez-Hernandez et al., 2018).
CO2 treatments have beneficial effects to control decay (Becatti et al., 2010; Blanch et al., 2012; Dong et al., 2015; Vazquez-Hernandez et al., 2018) without affecting the soluble solids content (SSC), titratable acidity (TA) or consumer acceptability (Blanch et al., 2012); increase the synthesis of skin monomers and oligomeric flavan-3-ol in grapes for regulating decay by Botrytis cinerea (Becatti et al., 2010); and reduce the activity of polyphenol oxidase and phenylalanine ammonia lyase and the synthesis of phenolic compounds (Dong et al., 2015). In the tomato, CO2 treatment has been effective in inhibiting the discoloration and softening of fruit during ripening stages; in particular, it has been more effective at the breaker stage than the pink stage (Choi et al., 2007). Recently, CO2-treated cherry tomatoes have been shown to have improved quality and extended shelf life (Sangwanangkul et al., 2017). However, the influence of high CO2 treatment on the quality of tomato ‘Dotaerang’ fruits during storage at different temperatures is poorly understood. Additionally, there is no previous study on the effect of CO2 treatment on the quality change of pink- colored tomato ‘Dotaerang’ fruits at different storage temperatures.
ClO2 gas is created by a reaction of an acid with sodium chlorite, a salt-based chemical frequently used as a sanitizer. ClO2 is more versatile than chlorine because it can be applied in gaseous or liquid form. Gas sanitizers are preferred over liquids because they more effectively cover the surface of produce and are more easily permeable to wounds or stem scars. There are some reports regarding the ClO2 functional effects on the quality of fresh produce such as reducing enzymatic browning, delaying ripening, and retarding respiration and ethylene production (Sadeghi et al., 2020). ClO2 is used as a gas fungicide to sterilize foodborne pathogens in fresh produce, and its effect on the spoilage of tomatoes have also been evaluated (Park et al., 2018). In particular, short-term, high-concentration ClO2 gaseous treatment was found to be very effective at inactivating Salmonella bacteria in Roma tomatoes (Olanya et al., 2015; Trinetta et al., 2010). However, there is little information available on the effects of ClO2 gas treatment on decay and quality changes of pink-colored tomato ‘Dotaerang’ fruits. Therefore, the aim of this study was to investigate the effect of the short-term CO2 treatment and cold storage on prolonging the shelf life and to find out whether co-treatment of CO2 and ClO2 could synergistic effect on the quality of pink-colored tomato ‘Dotaerang’ fruits during distribution period after harvest.
Materials and methods
Pink-colored tomato ‘Dotaerang’ fruits were collected at the breaker stage from the Agricultural Produce Center in Buyeo, Korea, in June 2019. Fruits were sorted according to maturity and size (diameter: 8-10 cm, weight: 200-240 g) immediately when they arrived at the National Institute of Horticultural and Herbal Institute. Fruits were placed in commercial cardboard boxes (Total weight of tomato: 4 kg, 10-12 fruits) and covered with plastic film for control (convention), 30% CO2, 60% CO2, or 30% CO2 combined with 10 ppm ClO2 (mixed with ambient air) treatment. For CO2 treatment, tomato boxes were put in an acrylic chamber and subjected to a 3 h CO2 treatment. ClO2 treatment was prepared using a ClO2 gas generating system (CA300, Purgo Farm, Hwaseong, Korea). Ten ppm ClO2 was used as referred by Choi et al. (2013) studied the effects of ClO2 treatments on the quality parameters of tomatoes, such as firmness, weight loss, gas concentration, color, and decay rate, during storage at low temperature (5°C) and room temperature (23°C). ClO2 concentration was treated as a single concentration to determine the effect of the CO2 and ClO2 combination treatment. The CO2 concentration in the chamber was verified by a portable headspace analyzer (CheckPoint II, Dansensor, Ringsted, Denmark). After the CO2 and/or ClO2 treatment concluded, treated tomato boxes were covered with plastic film and kept at 4°C (cold storage) and 20°C (room temperature storage) to see whether those treatments improve the fruit quality including chilling injury. In addition, the temperature were set at 4°C during transportation when tomatoes were exported by ship. In case of them, the chilling injuries were often occurred, In this study, to develop technology to suppress chilling injury during distribution. CO2 and/or ClO2 treated tomatoes which were stored at 4°C, fruit quality and ethylene production rates were assessed for 14 days and/or transferred to 20°C for 3 days (14+3 days). The reason for 14 days and/or transferred to 20°C for 3 days was to investigate the quality change during distribution at room temperature after low temperature transportation. Tomatoes stored at 20°C were subjected to assessment of quality factors and the ethylene production rate for 13 days. In all analysis results, 0 days represent the value after treatment and before storage.
Respiration and ethylene production were analyzed with a gas chromatograph (GC 7890B, Agilent Technology, Santa Clara, CA, USA). In a 3.4 L sealed container containing 3 fruits, 1 mL of gas was collected with a syringe after 2 h of tomato ethylene production. Three replicates were measured per treatment. The injection and column temperatures were 110°C and 70°C, respectively. The thermal conductivity detector and flame ionization detector used for respiration and ethylene measurements were set to 150°C and 250°C, respectively.
The firmness of fruit (10 tomatoes with three replicate per treatment) was measured with a texture analyzer (TA Plus, Lloyd Instruments Ltd., Fareham, Hampshire, UK) at a rate of 2 mm/s using a 5 mm diameter flat probe. SSC was determined using a digital refractometer (PAL-1, Atago, Japan). TA was determined by titrating 5 mL of juice with 0.1 N NaOH to pH 8.2 using an auto pH titrator (TitroLine easy, SI Analytics GmbH, Mainz, Germany), and expressed in grams of citric acid per 100 g of sample juice. For the SSC and TA, nine fruits were measured per treatment, and three fruits were juiced per replication and measured in three replicates.
Fruit surface color was measured using a colorimeter (CR-400 Minolta Chroma Meter, Konica Minolta Sensing Inc., Tokyo, Japan) and reported using Hunter values (lightness (L*), redness (a*), and yellowness (b*)). Ten tomatoes per treatment were measured, with three readings on three opposite sides of the equatorial region. Extracted lycopene content of tomatoes was determined with a modified colorimetric method (Chang et al., 2006). Tomato samples were freeze-dried and then ground with a commercial blender (SMX-S200SH, Shinil, Cheonan, Korea). Extraction was carried out by mixing 0.1 g of freeze-dried sample powder with 15 mL of an organic solvent mixture of hexane, methanol, and acetone at a 2:1:1 ratio. The procedure used an incubator shaker (NB-205QF, N-Biotech, Hwaseong, Korea) at 300 rpm and 25°C for a 1 h extraction. The extracted solution was centrifuged at 15,000 ×g for 10 min and then filtered with an 0.5 μm syringe filter. The resulting absorbance of the extract at 502 nm was measured using UV/vis against the blank extract solvent, and lycopene concentrations were calculated using the extinction coefficient (E %) of 3150.
CI was measured as described by Park et al. (2018): 0=no pitting, 1=few scattered pits, 2=pitting covering up to 5% of the fruit surface, 3=pitting covering 5 to 25% of the fruit surface and 4=extensive pitting covering >25% of the fruit surface. Fruit decay was determined in each treatment at the end of 14+3 days (cold storage) or 13 days (room temperature storage) and expressed as the decay percentage. Fruit decay was expressed as the percentage of fruit showing any decay symptoms. The CI index and decay rate were taken from three replicates (3 fruits per replicate) per treatment per day. Electrolyte leakage (EL) was determined as described by Li et al. (2011). To measure EL, 6 flesh disks (5 mm thickness × 10 mm diameter) from the flesh tissue of fruit were collected with a cork borer. Fresh tissue was collected from three fruit for each treatment. Disks were immersed in 50 mL of doubled distilled water for 1 h. The initial conductivity of the sample solution was determined with a conductivity meter (Orion Star, Thermo Fisher Scientific, Waltham, MA, USA), the disk solution was boiled for 30 min and cooled at room temperature, and the final conductivity was measured. EL was expressed as relative conductivity (the initial conductivity/the final conductivity).
Tomato samples for antioxidant analysis were freeze-dried and then ground with a commercial blender (SMX-S200SH, Shinil). Thereafter, 1 mL of 70% methanol was added to 0.1 g of freeze-dried sample powder, and the sample was extracted at 300 rpm and 25°C for 1 hour using an incubator shaker (NB-205QF, N-Biotech). The extract solution was centrifuged at 15,000 g for 10 min and then filtered with a 0.5 μm syringe filter. The total phenolic compound concentrations of tomato extract were measured with the Folin-Ciocalteu colorimetric method. For the test solutions, 100 μL of 1 N Folin-Ciocalteu reagent was added to 100 μL of tomato extract or standard. This was placed in the dark for 3 min at room temperature. Finally, 1 mL of 2% Na2CO3 was added, and the sample was placed in the dark for 30 min at room temperature. Once the test solutions had completely reacted, absorbance at wavelengths of 726 nm was measured using a spectrophotometer. Gallic acid was used as a standard to produce a reference standard curve with solutions prepared to concentrations of 100, 200, 300, and 400 mg/L. The total phenolic compound concentrations were expressed as milligrams of gallic acid equivalents per 100 g dry weight (DW). The 1,1-diphenyl-2-picrylhydrazyl (DPPH) radical scavenging activity of the samples was evaluated according to the method developed by Lee et al. (2012). The solutions were prepared by mixing 20 μL of tomato extract and 180 μL of 0.36 mM DPPH solution, and the mixtures were allowed to react for 20 min in the dark at room temperature. Absorbance at 515 nm was measured using a spectrophotometer. Trolox was used as a standard to produce a reference standard curve with solutions prepared to concentrations of 200, 400, 600, 800, and 1,000 μM. The DPPH radical scavenging activity of tomato extracts was expressed on the DW basis as μmol of trolox equivalents per g DW. The 2,2-azino-bis-3-ethylbenzothia-zoline-6-sulfonic acid (ABTS+) radical scavenging activity of the samples was evaluated according to the method developed by Lee et al. (2012). ABTS stock solution (ABTS+ solution) was prepared from 7 mM ABTS and a 2.45 mM potassium persulfate solution and kept in the dark for 16 h. The solutions were prepared by mixing 20 μL of tomato extract and 180 μL of the ABTS+ solution, and the solutions were allowed to react for 7 min in the dark at room temperature. Absorbance at 734 nm was measured using a spectrophotometer. The ABTS radical scavenging activity of tomato extracts was expressed on the DW basis as μmol of trolox equivalents per g DW. Samples of each extraction were analyzed in triplicate.
Each test was replicated three times and assessed using analysis of variance (ANOVA) in the SAS statistics program (SAS Institute, Cary, NC, USA) and SPSS program (SPSS Inc., Chicago, IL, USA). Significance was analyzed via Duncan’s multiple range test (p≤0.05), and correlations among antioxidant compounds and activity were analyzed using Pearson’s correlation coefficient.
Results and discussion
The results of the respiration rate and ethylene production analyses of tomatoes under short-term CO2 treatment are shown in Fig. 1A and 1B, respectively. At day 0, the difference between respiration rate and ethylene production was judged to be due to sample differences, not the effect of treatment. High CO2 treatment inhibited respiration and reduced ethylene production during the cold storage; however, there was no significant difference among treatments. The 30% CO2-treated tomatoes showed the least amount of respiration compared to those in other treatment groups and similar ethylene production trends. In cold storage, it seems that the lowering of the respiration rate and ethylene as the storage period elapses is the cold storage effect rather than the treatment period.
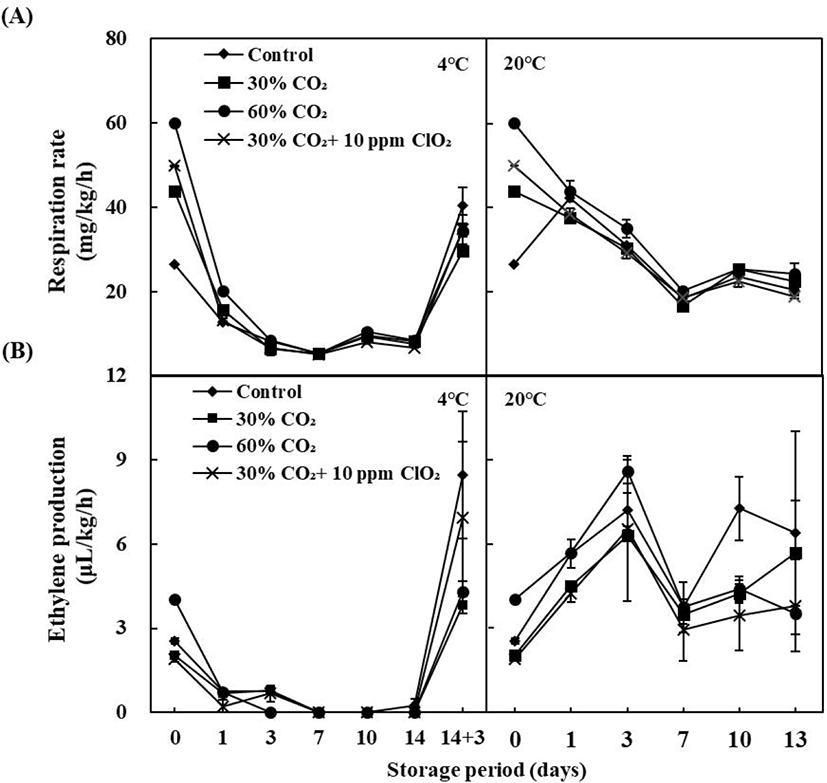
At room temperature, the respiration rate from all treatments decreased sharply until 7 days of storage, and then the rate stabilized until the end of the experiment (Fig. 1A). Although the rate of ethylene production fluctuated across the 20°C storage period, the highest and lowest rates were observed at 3 days and 7 days of storage, respectively (Fig. 1B). At 10 days of storage at 20°C, the high CO2 treatment group had a low ethylene production rate compared to the control group. Among the different storage temperatures, the rate of respiration and ethylene production from samples in cold storage were lower than those of samples in room temperature storage. The pattern of respiration and ethylene production is a climacteric type, and it is apparent at room temperature storage because tomatoes are metabolically active and their quality changes rapidly due to high oxygen consumption under adverse conditions. According to this result, low-temperature storage was effective methods to suppress respiration and maintain pink-colored tomato ‘Dotaerang’ fruit storage quality and shelf life.
There was no significant difference between CO2 treatment and the combination treatment of CO2 and ClO2 in respiration rate and ethylene production. Previous studies have reported that respiratory and metabolic activity in harvested climacteric fruits, particularly tomatoes, is directly related to ambient temperature. While the ethylene production rates in tomatoes were the highest at 20°C, the rate of respiration is directly proportional to the increasing temperature (Arah et al., 2015; Mutari and Debbie, 2011).
The variation in the quality of tomatoes based on CO2 and/or ClO2 treatment and storage temperatures are shown in Table 1. During the distribution of tomatoes from farm to market, preventing softening is very important because fruit firmness decreases greatly. In this experiment’s finding, the initial firmness level of fruit from each treatment is control (13.50±0.48), 30% CO2 (12.75±0.48), 60% CO2 (13.19±0.66), and 30% CO2+10 ppm ClO2 (13.95±0.59). By treating with CO2 and/or ClO2, treated sample groups such as 30% CO2 (7.14±0.36), 60% CO2 (6.08±0.31), 30% CO2+10 ppm ClO2 (6.90±0.26) were firmer than the untreated sample-control (5.72±0.2)-after storage at 4°C for 14 days and/or transferred to 20°C for 3 days (14+3 d). Although a longer duration of storage tended to lower firmness, storage at room temperature resulted in a loss in fruit firmness; in particular, there was a two-fold decrease in firmness of fruit at room temperature compared to that in cold storage at the one week assessments (Table. 1). At room temperature storage, there was no significant difference between treatments. This result indicated that low temperature and CO2 and/or ClO2 treatments effectively inhibited the softening of pink-colored tomato ‘Dotaerang’ fruits because CO2 treatment affects the subsequent precipitation of soluble pectin and the enhancement of cell-to-cell bonding (Harker et al., 2000). Sadeghi et al. (2020) found that the firmness of cherry tomatoes treated with ClO2 gas was maintained during the storage time compared to that of the control samples, which was similar to the results of this study. Vazquez-Hernandez et al. (2018) also found that CO2-treated grapes maintained their firmness during the storage period. SSC were not influenced by CO2 and/or ClO2 treatment. TA decreased more in room temperature storage than in cold storage (Table 1). Previous studies have shown that changes in SSC and SSC/TA ratios result from normal metabolic activity after harvest, and cold storage effectively extends shelf life by delaying metabolic activities (Tano et al., 2007; Vazquez-Hernandez et al., 2018).
By comparing the treatment and storage temperature, the fruit surface color of tomatoes is shown in Fig. 2B. Fruit surface color is one of the most important visual characteristics comprising the ripening index of tomato. In our results, cold storage and CO2 and/or ClO2 treatment delayed fruit surface color development. Storage temperature significantly affected fruit redness (a*). The a* values of all tomatoes increased during storage, although these values were always significantly lower in tomatoes stored at 4°C than those stored at 20°C after the shelf-life period. At 7 days of storage at 4°C, the a* values of control (4.37), 30% CO2 (0.19), 60% CO2 (0.52), and 30% CO2+10 ppm ClO2 (0.66) treatment were lower than the control (18.68), 30% CO2 (19.59), 60% CO2 (19.19), and 30% CO2+10 ppm ClO2 (19.38) after 7 days of storage at 20°C (Fig. 2B). CO2 treatments affect fruit surface color; however, there was no effect of CO2 and/or ClO2 treatment in storage at 20°C. The a* values were significantly lower in CO2 and/or ClO2- treated tomatoes than those of the control group in storage at 4°C. Fruit surface color was affected by low temperature storage (Sangwanangkul et al., 2017); in particular, the high CO2 treatments with cold storage conditions were more impacted in fruit surface color retraction compared with the control. Deltsidis et al. (2011) found that the lower temperatures and elevated CO2 atmospheres seemed to act synergistically in delaying the ripening process. By comparing the treatment and storage temperature, the lycopene content of tomatoes is shown in Fig. 2C. At 14 days of storage at 4°C, the lycopene content of 30% CO2 (20.48±1.13 mg/100 g DW), 60% CO2 (22.48±1.44 mg/100 g DW), and 30% CO2+10 ppm ClO2 (22.43±0.19 mg/100 g DW) treatment samples showed significantly lower values than the control (30.05±1.72 mg/100 g DW) (p<0.05). The lycopene content of the CO2 treatment samples from 20°C storage, such as 30% CO2 (85.10±3.12 mg/100 g DW), 60% CO2 (93.27± 0.13 mg/100 g DW), 30% CO2+10 ppm ClO2 (84.19±2.18 mg/100 g DW), also showed significantly lower values than the control (102.57±3.42 mg/100 g DW) (p<0.05). Among the storage temperatures, lycopene content of room temperature tomatoes stored for 7 days was 6-8-fold higher than those stored in the cold for 7 days. Generally, the accumulation of lycopene content in fruit stored at 20°C is higher by at least 3-fold than that of 4°C storage fruit. Toor and Savage (2006) found that 12°C low temperature storage increase lycopene content and antioxidant activity in tomato rather than 5 and 7°C storage conditions. The correlations between fruit surface color and lycopene content at different storage temperatures are shown in Fig. 3A. High positive correlation coefficients (R=0.800 and 0.861, respectively) were found between a* value and lycopene content of tomatoes during storage at cold and room temperature. Batu (2004) also found that the a* value is a good parameter for judging red color development and the degree of ripening in tomatoes. Similarly, the results of this study also showed a tendency for the a* value to increase as ripening progresses. In this study, CO2 and/or ClO2 treatment and low temperature storage inhibited the accumulation of lycopene and the ripening process of pink-colored tomato ‘Dotaerang’ fruits, and this approach effectively maintains fruit freshness. Javanmardi and Kubota (2006) found that the accumulation of lycopene and the rate of ripening are bound together under the same environmental condition. Additionally, this study showed that low temperature storage at 4°C inhibited the ripening of tomatoes and that CA storage inhibited lycopene accumulation in tomatoes (Giovanelli et al., 1999; Sozzi et al., 1999).
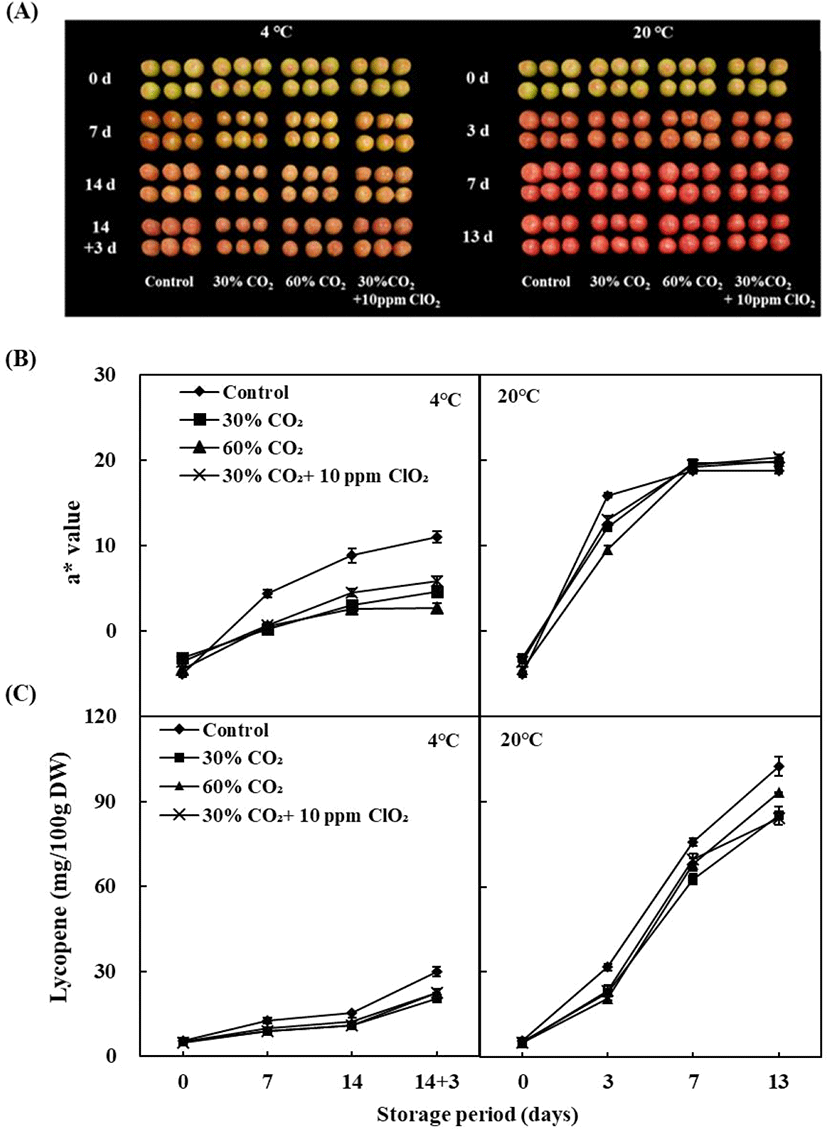
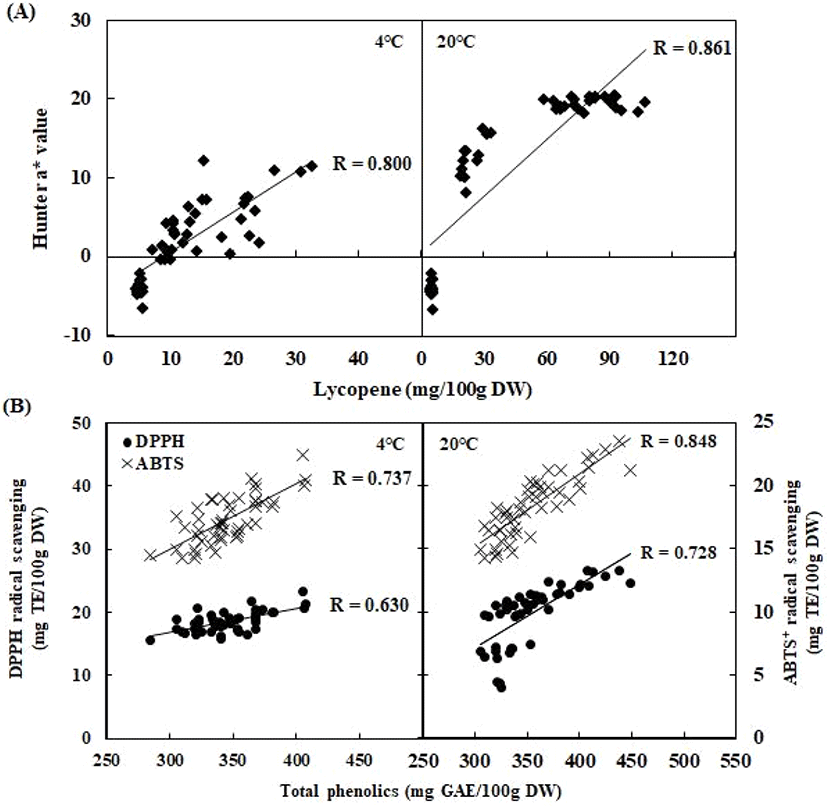
During the post-harvest operation in a cold-chain system, CI can occur due to the extrinsic factor of low temperature. To solve this problem, we examined the CI index of CO2 and/or ClO2-treated tomatoes during storage at 4°C for 14 days and/or transfer to 20°C for 3 days. In this study, the CI indices of high CO2-treated tomatoes were 30% CO2 (1.8) and 60% CO2 (1.6), which were lower than those of the control (2.3) after storage at 4°C for 14 days and/or transferred to 20°C for 3 days (14+3 days); however, 30% CO2+10 ppm ClO2 treatment was not significantly different from the control (Fig. 4A). According to these results, high CO2 treatment is effective at preventing CI during post-harvest cold- chain procedures. Some post-harvest technologies, such as controlled atmosphere, have also been shown to modulate membrane deterioration and prevent some physiological disorders related to texture caused by chilling temperatures (Maldonado et al., 2002; Sozzi et al., 1999). The degree of CI severity depends on intrinsic factors such as varieties, growth conditions, exposure to stress, and extrinsic factors such as temperature, relation humidity, and duration of stress exposure (Luengwilai et al., 2012). Aghdam and Mohammadkhani (2014) suggest that if fruits or vegetables are exposed to low temperatures for a long time, loss of cell membrane integrity leads to leakage of intracellular contents, which can be confirmed by measuring EL. The EL measurements in this study remained constant during cold storage (Fig. 4B). These results suggest that cold storage for 2 weeks does not significantly affect tomato quality. Post-harvest decay is important when considering the economic losses of the tomato industry. Incidences of tomatoes decaying in high CO2 and/or ClO2 treatments are shown in Fig. 4C. At storage at 4°C for 14 days and/or transferred to 20°C for 3 days (14+3 days), the decay incidence of 30% CO2 (33.5%), 60% CO2 (32.0%), and 30% CO2+10 ppm ClO2 (25.0%) treatment samples showed significantly lower values than that of the control (50.5%). The decay incidence of the CO2 treatments from 20°C storage was 30% CO2 (42.9%), 60% CO2 (44.3%), and 30% CO2+10 ppm ClO2 (26.0%), and they also were significantly lower than that of the control (58.6%) (p≤0.05). According to the results, low temperature storage more effectively inhibited decay than room temperature storage. CO2 gas treatment is a post-harvest technique that is widely used to control the decay of fruits and vegetables such as strawberry and tomato (Blanch et al., 2012; Sangwanangkul et al., 2017). Choi et al. (2013) found that ClO2 treatment is effective in maintaining firmness and soluble solids content because it decreases respiration, the total number of bacterial cells, and the rate of decay. ClO2 gas treatment may be useful to prevent fungal incidence as well as to retain the postharvest quality and increase the shelf life of tomato fruits. Additionally, in this study, 30% CO2 combined with 10 ppm ClO2 treatment were very effective in preventing decay of tomato ‘Dotaerang’ fruits during long-term storage at low temperature, which was consistent with the results of Ma et al. (2020) who reported that while CA treatments markedly delayed the mold development of fresh walnuts, a combination with ClO2 was the most efficient.
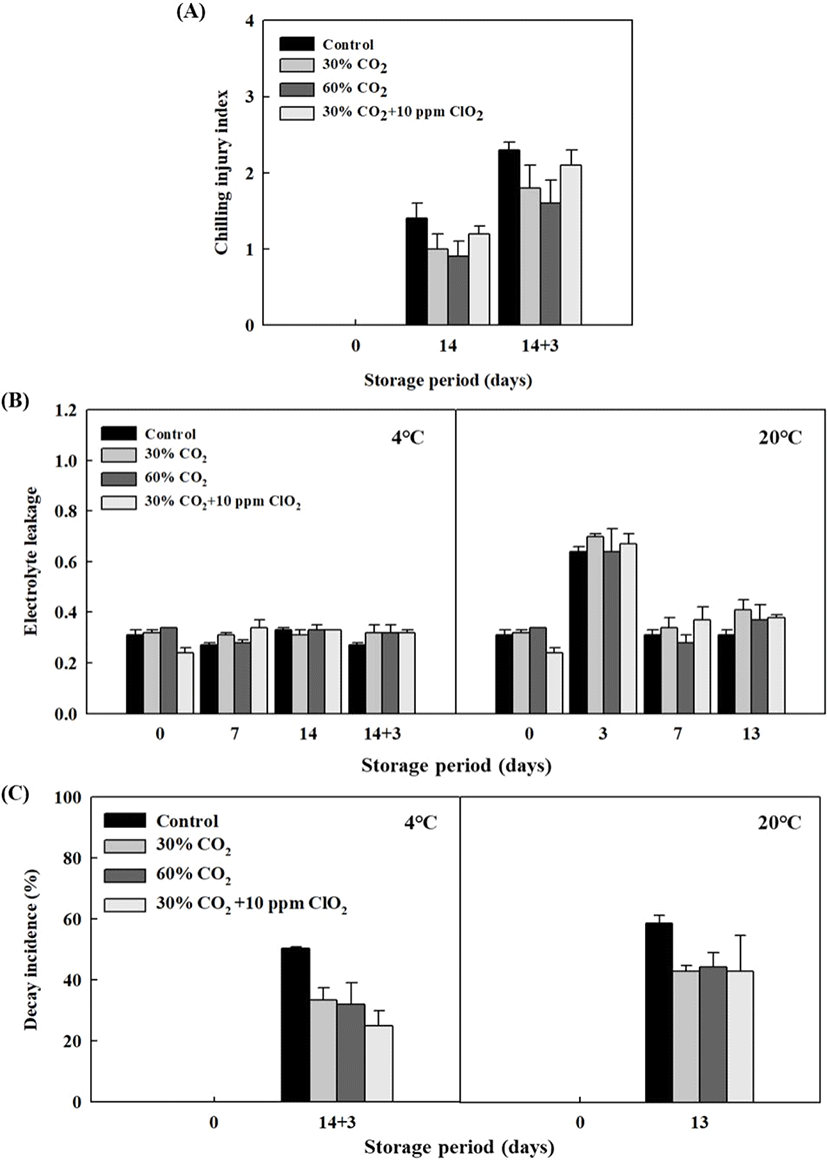
The total phenolics and antioxidant activities of tomatoes subjected to different CO2 and/or ClO2 treatments and storage temperatures are shown in Table 2. The total phenolics and antioxidant activities of tomatoes treated with CO2 and ClO2 increased with longer storage periods; however, there was no significant difference between treatments. Increasing antioxidant activity during storage could be related to ripening processes and the metabolism of phenolic compounds (Besada et al., 2015). After cold storage for 7 days, the total phenolic contents of 30% CO2 (325.71 mg/100 g DW), 60% CO2 (335.26 mg/100 g DW), and 30% CO2+10 ppm ClO2 (347.53 mg/100 g DW) samples was significantly lower than those of 30% CO2 (387.53 mg/100 g DW), 60% CO2 (361.36 mg/100 g DW), and 30% CO2+10 ppm ClO2 (414.09 mg/100 g DW) at room temperature storage for 7 days. DPPH and ABTS+ radical scavenging results showed a similar pattern to total phenolic contents. However, the control sample from cold storage was slightly higher than that from room temperature storage. In general, biotic and abiotic stresses lead to higher phenolic metabolism and antioxidant capacity (Olanya et al., 2015), and environmental stresses such as low temperature induce free radicals in plants (Hodges et al., 2004). The high levels of reactive oxygen species, produced during cold stress, have been closely related to the onset of chilling injury (Cai et al., 2006). However, in this study, cold storage slightly induces antioxidant activity, but applying CO2 treatment showed lower antioxidant activity than those of control. The correlations between total phenolic and antioxidant activities at different storage temperatures are shown in Fig. 3B. In both cold and room temperature storage, there was a somewhat higher and/or higher correlation between total phenolic and DPPH and ABTS+ radical scavenging. This is similar to the correlation result between the a* value and lycopene in this study, and antioxidant activities were also significantly higher as ripening progressed. Consistent with the results, Joradol et al. (2019) found that ClO2 fumigation triggered NOX-dependent H2O2 production to reduce peel browning and maintain fruit quality, thereby activating antioxidant reactions to overcome subsequent H2O2 production.