Introduction
Seaweeds are associated with Asian cuisine, especially in ready-to-eat food such as Kimbab (steamed rice rolled in dried laver) and sushi. The health benefits of seaweed consumption have been demonstrated in numerous clinical studies (1,2). Laver (Porphyra spp.) is one of the most important seaweeds and classified in the Bangiaceae, Bangiophycidae, Rhodophyceae and Rhodophyta families. Asian countries have been cultivating laver for many years.
In particular, Korea, Japan, and China are the world’s major areas of laver production (3). Of the several varieties the major species of laver cultivated in South Korea are Porphyra. yezoensis Ueda, P. tenera Kjellman, P. seriata Kjellman, and P. dentata Kjellman (4), and all grow well in seawater. Cultivated lavers are normally consumed in dried form, and several products are also being made. Depending on the raw materials the products are mainly classified into three major groups such as traditional laver (Gim), stone laver (Dolgim), and green laver (Paraegim). The contents of the traditional lavers are P. yezoensis Ueda and P. tenera Kjellman. In contrast, stone lavers have a smooth texture because of the presence of 30-40% of green algae. They are mainly composed of P. seriata Kjellman and P. dentata. On the other hand, the green laver is known as Aonori in Japan and Parae in Korean and is a type of edible green seaweed-including species from the genera Monostroma. Various small manufacturers are now involved in the preparation of dried lavers having low moisture contents (typically less than 10%). However, a major concern is that the prepared laver products are vulnerable to various microorganisms, resulting in concerns over hygiene. Choi et al. (5) reported that the dried laver had a high initial aerobic plate count (APC) level (4.4 to 7.8 log CFU/g). In addition, the presence of Coliforms and Bacillus cereus have been observed in some raw materials and semi-processed products. Therefore, it is necessary to ensure that the dried laver is prepared with good hygienic practices and are safe for consumption.
One promising approach to decontaminate laver from microbes is radiation processing. In comparison with thermal treatment, the radiation processing of food and associated products has been shown to be effective in reducing bacterial levels. Furthermore, radiation processing does not leave any chemical residues in the food products (6). A maximum radiation dose of 10 kGy has been reported to be effective for seaweed preservation (7). However, the employment of this technology is regulated by various national and international guidelines and has mandatory labeling requirements. Therefore, authentic detection methods are of paramount importance to enforce the regulatory guidelines and to enhance consumer acceptance of irradiated food commodities (8). A significant improvement has been made in the field of detection of irradiated food in recent years (9,10), and several international standards have been established. The European Standardization Committee (CEN) has set standards for detection of irradiated foods and identified electron spin resonance (ESR) spectroscopy as a unique non-destructive technique to detect radiation-induced paramagnetic centers in food commodities. The Codex Alimentarius Commission has also approved these international standards. Concerning ESR spectroscopic approaches, three standards have been established, EN 1786, EN 1787, and EN 13708, for food materials comprising of bone, cellulose, and crystalline sugars, respectively (11-13). However, the presence of high levels of moisture in majority of the food commodities prevents the detection of these radicals because of their limited lifetimes (14). In addition, the interactions between different forms of ionizing energy and biological materials are complex in nature. The interactions are influenced by the applied irradiation dose and post-irradiation storage conditions. Consequently, the detection of irradiation has become an intriguing task with several challenges. To address these issues, several different sample pretreatment steps to improve the detection of radiation-induced signals have been investigated and reported in literature such as freeze-drying (13), oven-drying (15) or other techniques (16). In our recent communication (17), the influence of different sample pretreatments (freeze drying (FD), alcoholic extraction (AE), NaOH extraction (NE) and KOH extraction (KE)) on the paramagnetic characteristics of different sea algae after irradiation was studied using ESR spectroscopy. In case of seaweed a new radiation-induced paramagnetic center with a hyperfine coupling (hfcc) of 2.3 mT was detected after NE and KE. However, the origin of the radiation-induced paramagnetic centers was not identified. On the other hand, AE was found to be an appropriate approach to detect the radiation-induced cellulose signal in irradiated sea mustard. It was observed that the suitable post-irradiation sample pretreatments of seaweeds could be a promising approach to detect irradiation history.
The objective of this study was to detect and understand the origin of radiation-induced signals in irradiated laver seaweeds by improving ESR spectral features through several post-irradiation pretreatment steps. An investigation was also made to identify the source of the paramagnetic centers by studying post-irradiation behavior of some chemical components associated with the biological composition of the samples.
Materials and methods
Dried traditional laver, stone laver, and green laver products were procured from a local market in Daegu, Korea and stored at room temperature. Glycine, alanine, and cellulose were procured from Sigma as standard materials to study the ESR responses after exposure to radiation and heat. The samples were irradiated at 7 kGy with an electron beam generated using a linear accelerator (ELV-4, 10 MeV, Fujifilm, Tokyo, Japan) belonging to EB-Tech Co. (Daejeon, Korea). Alanine dosimeters (pellets) of 5 mm diameter (Bruker Co., Rheinstetten, Germany) were used to measure the applied dose, and a Bruker EMS 104 ESR analyzer (Bruker Co., Rheinstetten, Germany) was employed for dosimeter measurements.
Before ESR spectroscopic analysis, three different sample pretreatments were employed:
ⅰ) FD: Freeze-drying (Bondiro, Ilshinbiobase Co., Yangju, Kyunggido, Korea) of dried laver (12).
ⅱ) KE: Alkali hydrolysis extraction of the dried laver with 80% alcoholic KOH for 5 min (minute) using a sonicator, and the residues were used after drying with alcohol (18).
ⅲ) NE: Alkali hydrolysis extraction of the dried laver with 5% NaOH for 5 min using a sonicator, and the residues were used after drying with alcohol (17).
Both the alcoholic pretreatments were exothermic in nature, and the temperature of the samples increased to during treatment. To study the stability of the radiation-induced ESR lines at elevated temperature, irradiated standard materials (glycine, alanine, and cellulose) were also heated at 50, 100, and 150℃ for 30 min before the ESR measurements.
The samples were subjected to post-irradiation pretreatment and around 0.1 g of each was placed in a quartz ESR tube (5 mm diameter). The tube was sealed from the open end using plastic film. ESR spectroscopy was carried out in accordance with European standard, EN 1787 (12) using a X-band ESR spectrometer (JES-TE200, Jeol Co., Tokyo, Japan) at room temperature (20±2℃). The experimental parameters were as follows: power, 0.4 mW; frequency, 9.10-9.21 GHz; center field, 324±2 mT; sweep width, 10-25 mT; modulation frequency, 100 kHz; modulation width, 1-2 mT; amplitude, 50-400; sweep time, 30 s; and time constant, 0.03 s. The spectra were recorded in triplicate (n=3), and the mean values with standard deviation are reported. Microsoft Excel (Microsoft Office 2010) and Origin (version 8) software were used for data analysis and presentation. The computation of the height of the ESR signal was carried out by ESPRIT-425 software (Jeol Co., Tokyo, Japan). The signal height was calculated as the peak-to-peak amplitude the first-derivative spectrum, whereas arbitrary units are used for the presentation of the signal intensity. The g-values were determined using a standard 2,2-diphenyl-1-picrylhydrazyl (DPPH) sample with g = 2.0032. In addition, g-values were also confirmed by calculation using the relationship g = 71.448×microwave (GHz)/magnetic field (mT). This relationship was obtained by substituting the values of Bohr magneton (βe) and Planck’s constant (h) in appropriate units into the ESR resonance equation.
Results and discussion
Fig. 1 shows the ESR spectra of the dried lavers before and after exposure to ionizing radiation after the various sample pretreatments. The unirradiated samples were characterized by a singlet at g = 2.005, and this was attributed to photo-oxidation of the phenolic compounds present in the dried laver. This observed g-value was found to be in agreement with those reported in the published literature (10,19,20). Based on the available reports, these free radicals originate from semiquinones, which are produced by the oxidation of phenolic compounds (21) or lignin (22,23) in plants. The irradiated samples prepared after FD pretreatment exhibited an enhancement in the signal intensity of the existing central line at g = 2.005 (Fig. 1a). No new radiation-induced paramagnetic center was observed after FD pretreatment. The relatively high intensity was attributed to the irradiation treatment and many researchers have reported similar observations (24, 25). The irradiated laver samples with KOH extraction (KE) also did not exhibit any radiation-specific ESR lines (Fig. 1b) but, unlike the FD samples, the central ESR signal showed relatively decreased intensity at g = 2.005, probably because of the elimination of the radiation-induced polyphenol radicals because of alkaline hydrolysis. This observation is consistent with the results of our recent communication (17) where seaweed samples were exposed to different irradiation doses and the KOH pretreated samples exhibited a weak triplet signal at 10 kGy. On the other hand, in the case of the irradiated laver samples subjected to alkaline pretreatments with NE, a weak radiation-induced triplet was observed at the highest dose of 7 kGy (Fig. 1c). This signal is characterized by g = 2.006 and a hfcc of 2.3 mT. The spectral shape of this unknown signal is similar to that of the cellulosic radicals, and we have reported similar observations in our earlier communications (17). Normally, ESR signals in irradiated plant food containing cellulose include a pair of lines left and right of a non-specific central signal. This pair of lines is believed to be due to cellulose radicals formed by irradiation and is recognized as a marker for radiation treatment (12). The spacing of this radiation-induced signal pair is about 6 mT (hfcc of 3 mT). However, for irradiated dried laver, the hfcc (2.3 mT) was lower than that of the well-characterized radicals normally observed in irradiated foods of plant origin. Alkaline treatment with NaOH following irradiation resulted in a more prominent ESR triplet at 7 kGy; thus, NE is a potential treatment for the identification of irradiated dried laver, but the identification of this new ESR signal is an interesting task.
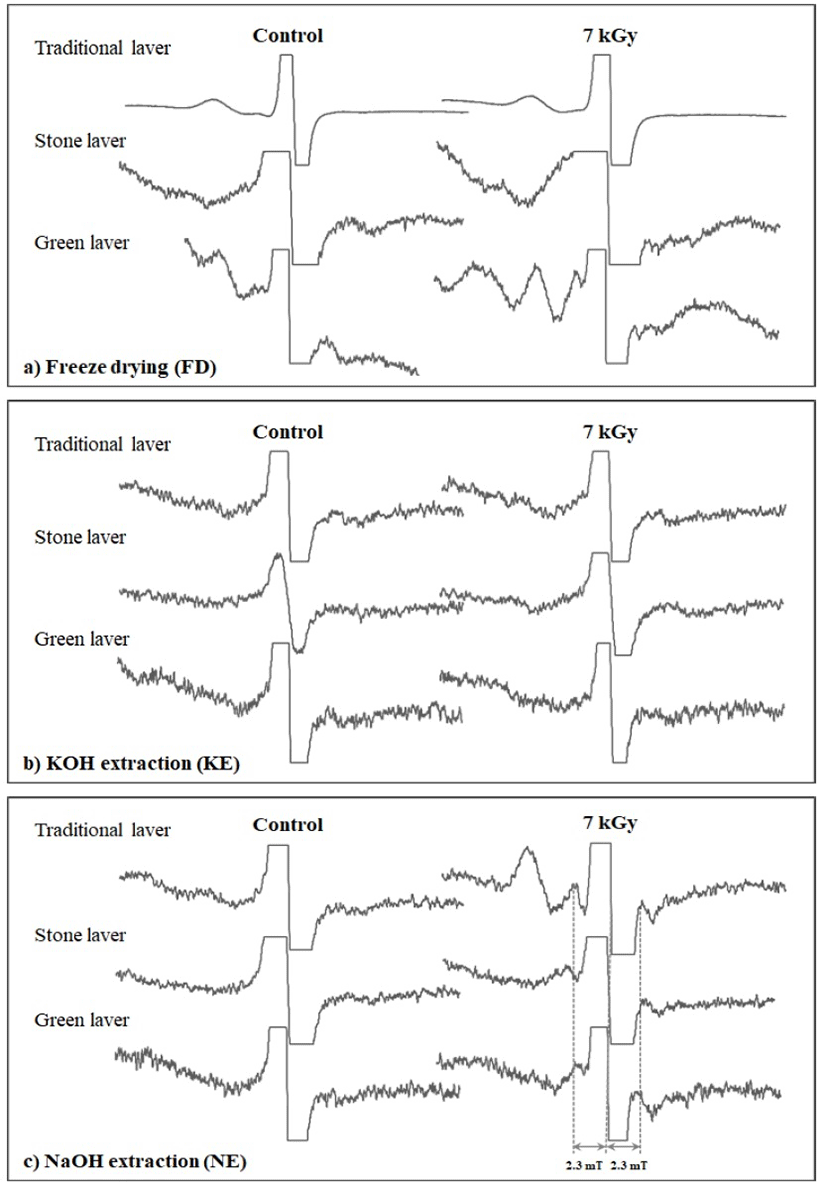
Laver has been recognized as a rich source of plant proteins (26), of which amino acids are the main building blocks. To understand the origin of the modified radiation-induced ESR signal in the treated dried laver, the ESR characteristics of the pure amino acids were studied after irradiation. In view of the triplet nature of the radiation-induced signal, the paramagnetic character of irradiated pure cellulose was also investigated. The amino acids (glycine and alanine) were irradiated with the same 7 kGy dose as that of the dried laver. The irradiated laver samples were subjected to alcoholic treatments before ESR measurements to enhance the visibility of the radiation-induced signals. Both NE and KE pretreatments involved an increase in temperature to 60°C. Consequently, the pure chemicals were also subjected to post-irradiation heat treatments to observe the changes in the shapes of the ESR signals. Fig. 2a shows the ESR spectra of the irradiated glycine before and after heat treatment. All the irradiated spectra exhibited complex signals, possibly because of the simultaneous trapping of several free radicals regardless of irradiation. In general, although amino acids are simple chemicals, they undergo complex radiation–chemical interactions, and, apparently, the nature of the side chains is crucial in determining the ultimate radical products detectible by ESR spectroscopy (27). ESR measurements of the simplest amino acid glycine reveal a triplet ESR signal after irradiation. However, the ESR spectrum of irradiated glycine is not simple and is highly dependent on the crystalline form (polymorphism) (28). Three different crystal polymorphs have been reported for glycine: the α-form, which is obtained by crystallization of glycine from aqueous solution, the β-form, which is obtained by facilitating crystallization by adding ethanol to a hot saturated aqueous glycine solution, and the γ-form, which is obtained by crystalizing glycine from a saturated aqueous solution of glycine with aqueous acetic acid (29). The crystal structure dependency on the free radicals found in irradiated glycine has been extensively studied, and the crystal structures of all these polymorphs are known. The triplet structure of the ESR spectrum of irradiated glycine in the α- and β-forms has also been well-established to have a hfcc on the order of 2 mT (29). However, the ESR spectra of the irradiated pure polycrystalline glycine samples obtained in our experiments were mainly dominated by the γ-form, as characterized by the main central ESR line at g = 2.006 with a total of five satellite lines. The left and right satellites around the central line have hfcc values on the order of 2 mT. However, the overall shape of the irradiated spectrum is different to those of the irradiated laver samples. To study the stability of the radiation-induced ESR lines upon heating, ESR spectra of the irradiated samples were recorded after heat treatment at 50, 100, and 150℃, as shown in Fig. 2a. All the ESR lines were observed to be quite stable with increasing temperature and the five-line structure was even visible at 150℃. Therefore, it is possible that the triplet of the irradiated laver samples did not originate from the radiation-induced paramagnetic centers of glycine. Irradiated alanine has a complex ESR spectra containing five lines at room temperature (30). Fig. 2b shows the ESR spectra of the irradiated alanine before and after heat treatment. The characteristic five-line structure of most stable alanine radical was observed having its central line at g = 2.005 with a hfcc of 2.1 mT. The central line and left and right satellite lines were similar to that of the irradiated spectrum of the laver. The stability of the extreme satellite lines with increasing temperature was studied to understand the role of alkaline hydrolysis, as shown in Fig. 2b. The signal intensity and visibility of all the ESR lines of irradiated alanine were stable with increasing temperature. The irradiated laver samples yielded ESR spectra having different final shapes. Thus, irradiated alanine was not responsible for the triplet signal in the irradiated laver. The strong stability of the satellite lines with increasing temperature in irradiated alanine has also been reported in the literature (31).
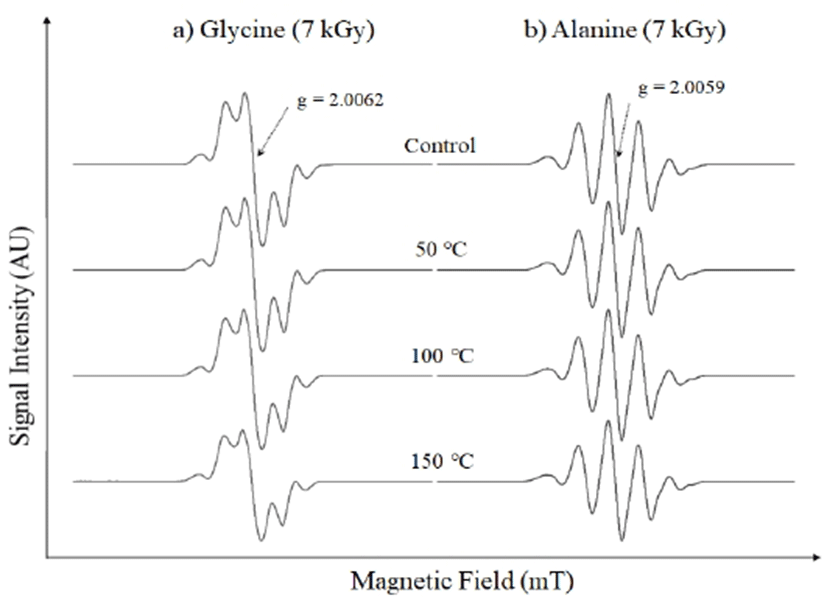
Like the amino acids, the pure cellulose was also irradiated and heat-treated to simulate the exothermic hydrolysis process and to understand its influence on the ESR spectral shapes. Fig. 3 shows the ESR spectra of the irradiated cellulose before and after heat treatments. The ESR spectrum of the irradiated cellulose without any heat treatment is complex in nature. The radiation-induced signals are characterized by a triplet at g = 2.015 with a hfcc 2.2 mT. In addition, another triplet was found to be superimposed at g = 2.005 with a hfcc of 3 mT, as shown in Fig. 3. Similar reports have been published with regards to irradiated cellulose where the ESR spectra of electron-beam-irradiated samples demonstrated complex characteristics showing the presence of multiple radical sites comprising of at least two triplets and one doublet with corresponding constants of 3.2, 2.2, and 2.0 mT, respectively (32). The monomeric ring of cellulose consists of five carbon atoms, as shown in Fig. 4 (32). The appearance of two triplet signals with corresponding hyperfine splitting of 3 and 2.2 mT could be ascribed to the formation of radicals at C-2, C-3, or C-4 (32). Normally, most irradiated foods exhibit a triplet signal of cellulose with a hfcc 3 mT (33). However, in the case of the irradiated laver, a triplet signal with a hfcc of 2.2 mT was prominent after alkaline hydrolysis pretreatment, possibly because the exothermic processes removed the cellulosic triplet (hfcc of 3 mT). To confirm this hypothesis, all the irradiated cellulose samples were subjected to heat treatments at 50, 100, and 150℃ before ESR measurements. The g-values of all the ESR lines with the respective magnetic fields with increasing temperature are shown in Table 1. As depicted in Fig. 3, the triplet at g = 2.005 fades with increasing temperature and disappeared at 150℃. However, the triplet at g = 2.015 (hfcc = 2.2 mT) was found to be quite stable with increasing temperature, showing a slight right shift of the central line from g = 2.015 to 2.006. In the case of the sample subjected to 50℃ heat treatment, a slight fading of the satellite lines was observed. On the other hand, the shape of the ESR spectrum of the irradiated cellulose heated at 100℃ was observed to be similar to that of the irradiated laver, having a triplet signal of hfcc of 2.2 mT. The ESR behavior of this cellulose sample is similar to that obtained after the alkaline hydrolysis of irradiated laver. Thus, it is proposed that the new radiation-induced signal in irradiated laver after chemical pretreatment is probably due to cellulosic radicals originating from different radical sites to those commonly observed.
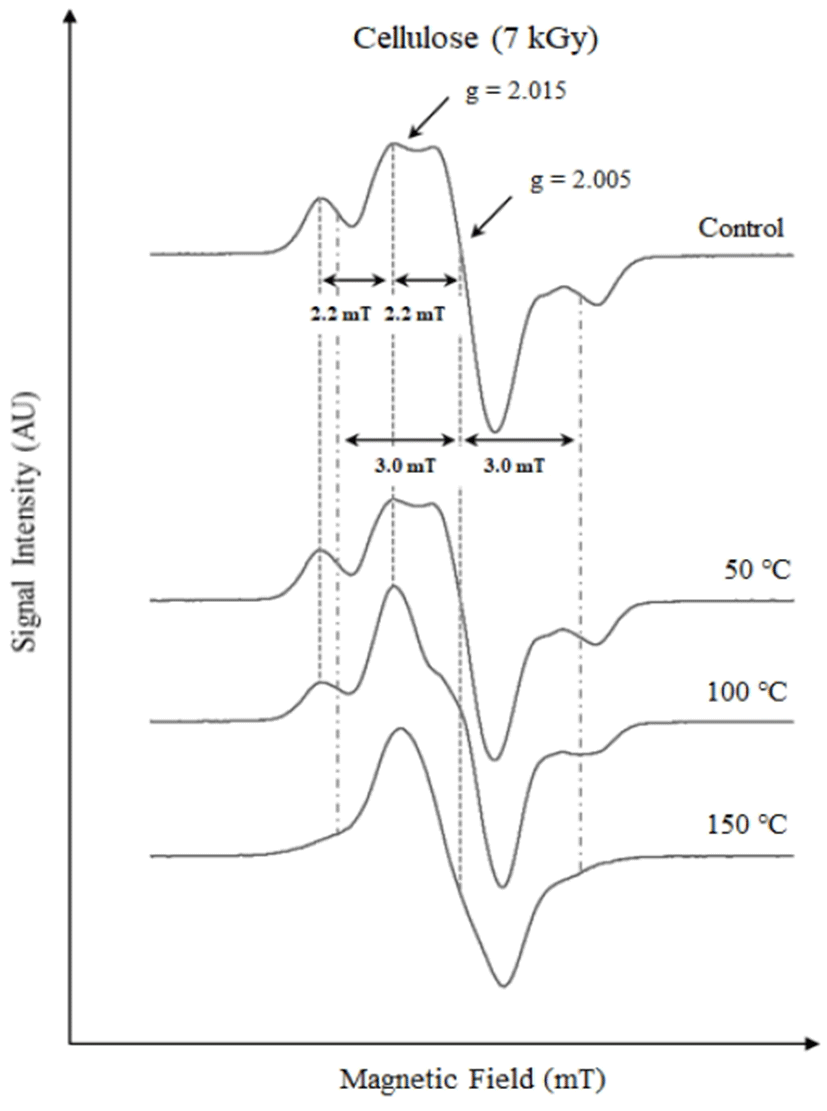
Conclusions
The identification of irradiated dried laver using ESR spectroscopy was improved using an alkaline extraction step prior to ESR measurements. Irradiated laver samples subjected to NE exhibited a radiation-induced triplet signal resembling that of cellulosic radicals. However, the ESR signal was characterized by a lower hfcc value in comparison to that of the standard cellulose radical signal that is usually observed in irradiated plant-derived foods. The ESR characteristics and thermal behavior of three irradiated pure chemicals (glycine, alanine, and cellulose) indicate that the new radiation-induced ESR signal in dried laver could originate from irradiated cellulose but from different radical sites to those commonly observed.