Introduction
Melanin is a dark-brown pigment that is synthesized in melanocyte of skin, hair and eyes. Melanin is one of the important factors that affect in skin color. Generally, melanin plays an important role in preventing the skin damage caused by ultraviolet (UV) (1). However, the abnormal accumulation of melanin pigment in the skin can lead to aesthetic problems, such as melasma, freckles, ephelides and senile lentigines.
The process of melanin synthesis is called melanogenesis and it starts with the oxidation of L-tyrosine. L-Tyrosine is oxidized to L-3,4-dihydroxyphenyl alanine (L-dopa) by tyrosinase enzyme and goes through dopaquinone and dopachrome, then finally forms melanin. Tyrosinase is an important enzyme in melanogenesis and melanogenesis is thought to be closely related with increase of tyrosinase activity (2). Thus, there has been many studies to search a potent tyrosinase inhibitor to regulate the skin hyperpigmentation, especially from the food-derived natural compounds (3).
Rutin (3-O-rhamnosylglucosyl-quercetin, Fig. 1A) is one of the biologically active flavonoids found in various foods and plants (4-6). After consumption, rutin is converted to various metabolites such as 3,4-dihydroxyphenyl acetic acid (DHPAA), 3-hydroxyphenyl acetic acid (HPAA), 3,4- dihydroxytolene (DHT) and homovanillic acid (HVA) (Fig. 1B, 1C, 1D, 1E) (7,8).
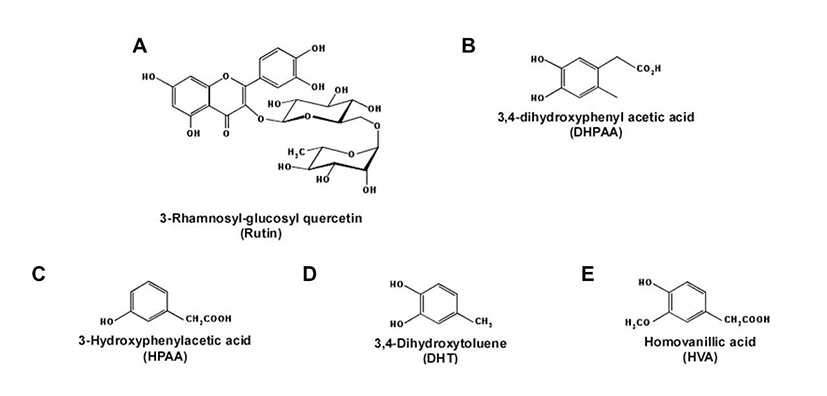
A variety of attention has been focused on the skin health promoting effects of rutin, including anti-oxidative (9) and anti-photoaging (10,11). Recently, rutin has been studied in terms of their ability to inhibit tyrosinase activity in vitro (12,13) and melanin production in the cell model system (14). In addition to rutin, its metabolites have reported to exert biological effects. Rutin metabolites inhibited the formation of advanced glycation end product on collagen in vitro (7) and this event may be due to the scavenging abilities against reactive oxygen species. Another study also reported that DHPAA and DHT protected from oxidative stress in the neuronal PC12 cells (15). However, there are no reports about the whitening effects of rutin metabolites, thus, we focused on the potential capacities of rutin metabolites.
Materials and Methods
Dulbecco’s modified Eagle’s medium (DMEM), penicillin-streptomycin and 0.5% Trypsin-EDTA were obtained from GIBCO® Invitrogen (Auckland, NZ). Fetal bovine serum (FBS), rutin, DPHAA, HPAA, DHT, HVA, mushroom tyrosinase (1,000 unit/mL), L-3,4-dihydroxyphenyl alanine (L-dopa) were purchased from Sigma-Aldrich (Sigma Chemical Co., St. Louis, MO, USA). 3-[4,5-dimethylthiazol- 2-yl]-5-(3-carboxymethoxyph enyl)-2-(4-sulfonyl)-2H-tetrazolium inner salt (MTS solution) was purchased from (Promega, Madison, WI, USA)
The JB6 P+ mouse epidermal skin cells were cultured in monolayers and incubated at 37°C in a humidified atmosphere containing 5% CO2 and 95% air. Cells were cultured in MEM containing 5% FBS, 2 mM L-glutamine and 100 μM penicillin/100 μg/mL streptomycin.
Cell viability was measured using the MTS assay. JB6 P+ cells were seeded (104 cells/well) in 96-well plates with 5% FBS, MEM at 37°C in a 5% CO2 incubator. After cell attachment for 6 hr, each sample was treated as indicated concentration (10, 20, 40 μM). After culturing for 24 hr, 20 μL of Cell Titer 96 Aqueous One Solution (Promega) were added to each well, and the cells were then incubated for 2 hr at 37°C in a 5% CO2 incubator. Absorbance was measured at 495 nm. Percentage cell survival was defined as the relative absorbance of treated versus untreated cells. Absorbance of each well was read at 495 nm with a microplate reader (Sunrise-Basic Tecan, Tecan Austria GmbH, 5082 Grödig, Austria).
Tyrosinase activity was assayed in terms of 3,4-dihydroxy phenylalanine (DOPA) oxidase activity, using a modified version of described method (16). Briefly, 80 μL of 0.1 M potassium phosphate buffer (pH 6.8) was placed on a 96-well plate. And then 40 μL of purified mushroom tyrosinase (1,000 unit/ml) and 40 μL of test sample was added at the indicated concentration (0, 20 or 40 μM). Then the microplate covered with aluminum foil was shaken at 23°C for 10 min. After shaking indicated time, 40 μL of 1 mM L-dopa was added into each well. The microplate covered 96-well plate with aluminum foil was shaken at 23°C for 10 min again. After shaking indicated time, the absorbance of each well was measured at 475 nm with a microplate reader (Sunrise-Basic Tecan, Tecan Austria GmbH). The value of each measurement was expressed as percentage changes from the control. Control wells contained in 40 μL 0.1 M potassium phosphate buffer (pH 6.8) and 40 μL of L-dopa.
Results and discussion
Tyrosinase is a copper containing oxidase and initial the rate-limiting enzyme for controlling the production of two types of pigments such as pheomelanin (red or yellow) or eumelanin (brown or black) (1). Tyrosinase catalyzes and involves the hydroxylation of L-tyrosine into melanin via L-dopa, dopaquinone, dopachrome and other intermediates (2,3). Therefore, suppression of the tyrosinase activity is considered as one of most effective strategy against skin hyperpigmentation.
Epidemiologic studies have shown that the various flavonoids in dietary foods may contribute to a reduced the risk of hyperpigmentation (17,18) and act as tyrosinase inhibitor in a competitive or uncompetitive manner (13). Especially, rutin and quercetin have reported to reduce mushroom tyrosinase activity (12,13). Interestingly, although rutin has no significant effects on melanin production in B16F10 melanoma cells (14), quercetin effectively attenuates the melanin production in B16 melanoma cells (14,19). However, there are no reports about the whitening effects of rutin metabolites compared to well-studied rutin or quercetin. In addition, it is uncertain whether quercetin is actual active compounds for whitening effect because it is also converted to metabolized forms (7). Therefore, we speculated the potential whitening capacities of rutin metabolites in this study.
We first evaluated whether rutin metabolites can affect the L-dopa oxidation in cell-free system using the mushroom tyrosinase inhibition assay. Mushroom tyrosinase is often used as a substitute for human tyrosinase in order to screen tyrosinase inhibitors because it is commercially available in a purified form (20,21). HVA had the highest anti-tyrosinase activity (51.0%), followed by DHPAA (53.3%), HPAA (57.8%), rutin (62.1%) and DHT (65.3%) at 40 μM, respectively (Fig. 2). Particularly, HVA at 20 μM exerts the significant inhibitory effect on tyrosinase activity, but other metabolites did not. Based on these results, IC50 values of each compounds were represented as Table 1 and showed consistent results with Fig. 2. Comparing with very well-known tyrosinase inhibitor such as arbutin (IC50 = 368.93 μM) (22), all of rutin metabolites showed much the lower IC50 values against mushroom tyrosinase activity. HVA (IC50 = 37.10 μM) possessed the most strong inhibitory capacity and other rutin metabolites also exhibited similar potency to kojic acid (IC50 = 40.69 μM) (22). These results revealed that all of rutin metabolites could be applied as a potent tyrosinase inhibitors.
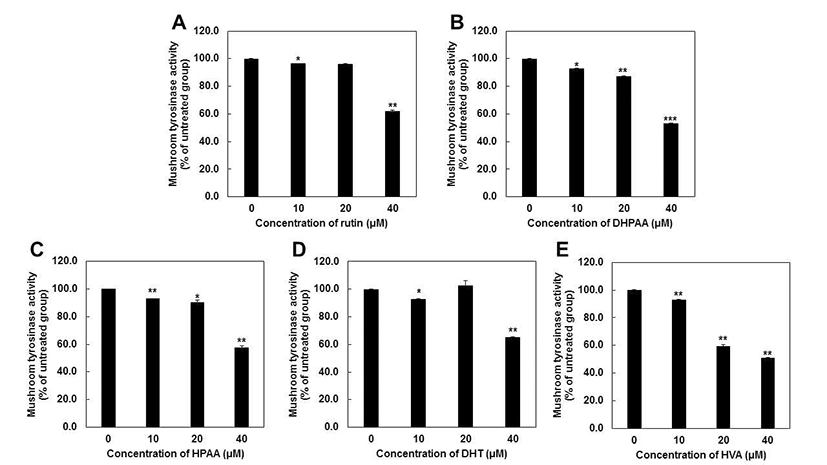
Group | IC50 values (μM) |
---|---|
Rutin | 57.98 |
DHPAA | 45.87 |
HPAA | 50.96 |
DHT | 66.09 |
HVA | 37.10 |
Although in this study, we used mushroom tyrosinase as a substitute for human tyrosinase in order to screen tyrosinase inhibitors, the use of mushroom tyrosinase is not sufficient because it is little different from human tyrosinase in terms of the amino acid sequence (23-25). In addition, some compounds that are active against mushroom tyrosinase do not show comparable results with mammalian tyrosinase (26-28). Therefore, for the further study, it is necessary to examine the inhibitory effects of rutin metabolites on human tyrosinase and human melanocytes.
Previous study reported that rutin or quercetin did not induce significant toxic effects up to 100 μM in various skin cells such as human fibroblast (10,11), HaCaT cells (29) and mouse melanoma cells (14,19). However, compared to rutin or quercetin, there are few studies about the effect of rutin metabolites on the proliferation of skin cells. Previous studies reported that DHPAA at 12 μM (30) and DHT at 100 μM (31) inhibited cell proliferation of B16F10 melanoma cells up to 50%. However, previous studies reported only one dose of concentration and other rutin metabolites, HPAA and HVA have not been elucidated their cytotoxicity. Thus, we evaluate the effect of rutin and rutin metabolites at various concentration from 25 to 100 μM in JB6 P+ mouse epidermal skin cells (Fig. 3).
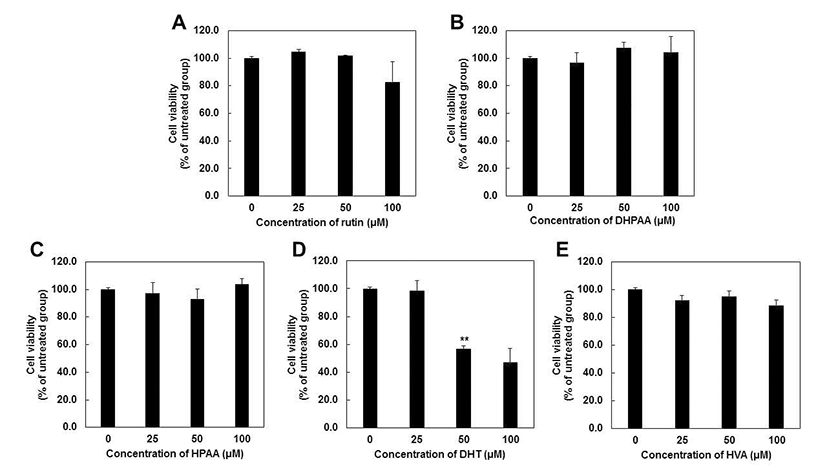
MTS assay showed that rutin and rutin metabolites including DHPAA, HPAA and HVA did not have cytotoxic effects at 24 hr after treatment at concentrations up to 100 μM (Fig. 3A, 3B, 3C and 3E). Unlike previous study (30), DHPAA did not exhibit cell cytotoxicity up to 100 μM. This is thought to be due to the different cell line and methods with previous study. DHT also was not toxic up to 40 μM (data not shown), but it showed the cell cytotoxicity at 50 and 100 μM by 56 and 47%, respectively (Fig. 3D). These results suggested that rutin metabolites were not toxic at 40 μM indicating that all of rutin metabolites can be applied as a potent tyrosinase inhibitor without cell cytotoxicity.
In summary, we first reported about whitening activities of rutin metabolites. According to our results, HVA was the most potent anti-tyrosinase inhibitor followed by DHPAA > HPAA > rutin > DHT. MTS assay results represented that, rutin and rutin metabolites had no effect on cell viability up to 100 μM except for DHT (up to 50 μM). Therefore, rutin metabolites could be suggested as a potential tyrosinase inhibitor and the whitening agent for the future.
요 약
본 연구는 rutin 및 rutin 대사체인 DHPAA, HPAA, DHT, HVA가 버섯 유래 tyrosinase 활성과 마우스 피부표피 세포 주인 JB6 P+ 세포의 증식에 미치는 영향에 대해 살펴보았 다. HVA가 버섯유래 티로시나제 저해활성이 가장 우수한 것으로 관찰되었으며, DHPAA, HPAA, rutin, DHT 순서로 저해효능을 나타내었다. DHT를 제외한 rutin 및 rutin 대사 체들 모두 100 μM 까지 세포 독성이 관찰되지 않은 것으로 관찰되었으며, DHT는 50 또는 100 μM에서 세포증식을 저해하였으나 그 이하의 농도에서는 세포독성을 유도하지 않았다. 따라서 본 연구결과, rutin 대사체 모두 세포 독성이 없는 수준에서 버섯 유래 티로시나제 활성을 효과적으로 억제하였으므로, 앞으로 미백제제로 활용될 수 있을 것으 로 기대된다.