Introduction
Obesity has become a serious global health problem in recent decades. Obesity can lead to many chronic diseases such as dyslipidemia, type 2 diabetes mellitus (T2DM), cardiovascular diseases, cancer and hypertension (1-5). Among them, T2DM is the most closely associated with obesity and they seem to share common causative factors, chemical abnormalities and clinical complications (6). Obesity is mainly caused by excessive food calorie intake and an abnormal accumulation of body fat (7). Consumption of a high-fat diet is a major risk factor that increases the chances of developing obesity and T2DM. Inhibition of digestive enzymes such as α-amylase, α-glucosidase and pancreatic lipase can decrease carbohydrate digestion and retard fat absorption, and therefore may be one of the most effective approaches to controlling obesity and related metabolic disorders.
Adipocyte, also known as fat cell, is associated with lipid homeostasis and energy balance (8). Therefore it has been widely used as an effective in vitro model for the evaluation of adipocyte differentiation. Adipocyte differentiation is the process by which undifferentiated preadipocytes are concerted to differentiated adipocyte. Adipocyte differentiation is regulated by two key transcription factors, peroxisome proliferator-activated receptor γ (PPARγ) and CCATT/ enhancer binding protein α (C/EBPα) (9). C/EBPα and PPARγ are activated during the differentiation process and promote together the expression of downstream adipose specific genes involved in adipose phenotype as well as in glucose and lipid metabolism (10,11). Sterol regulatory element binding protein-1c (SREBP-1c) is a well-known upstream regulator of PPARγ in the adipogenesis pathway (12). Moreover, SREBP-1c could regulate the genes involved in lipogenesis such as fatty acid synthase (FAS) and acetyl-CoA carboxylase (ACC) (13). Thus, controlling adipocyte differentiation has become a major target to prevent and treat obesity and related metabolic disorders.
The medicinal use of the root bark of mulberry has been documented in the Pharmacopoeia of People’s Republic of China and British Herbal Pharmacopoeia for a long history. Recently, it has been primarily used as antioxidant (14,15), antiasthmatic (16), antidepressant (17) and skin whitening ingredients (14). Besides, it was reported to have antiinflammatory and antiviral effects in vitro (18,19) and a hypolipidemic activity in vivo (15,20,21). Moreover, plants of this genus were known to be rich in flavonoids and prenylated flavonoids. The prenylated flavonoids such as morusin, kuwanon C, sanggenon D and kuwanon G isolated from mulberry root bark were reported to possess antioxidant, antimicrobial and anti-inflammatory activities (14,22,23). Recently, the phenolic compounds including maclurin, rutin, isoquercitrin, resveratrol and morin isolated from mulberry root bark showed potential antioxidant and whitening activities (24). It was reported that moracin M has a hypoglycemic effect on alloxan-diabetic mice (21). Additionally, morusalbanol A, a Diels-Alder type adduct with a new carbon skeleton, significantly attenuated the H2O2-induced cell damage and could be used as an euro-protective agent (25). However, to date, there have been no previous studies on the anti-adipogenic activity of mulberry root bark using 3T3-L1 cells and on the investigation of its molecular mechanism.
This study was aimed to investigate the inhibitory effects of mulberry root bark on digestive enzymes and 3T3-L1 preadipocytes differentiation. MRE was further evaluated for the potential mechanism underlying the regulation of cell differentiation, in which the gene expression patterns involved in adipogenesis and lipogenesis of 3T3-L1 adipocyte cells were analyzed.
Materials and methods
3T3-L1 cells were purchased from American Type Culture Collection (ATCC). Dulbecco’s modified Eagle’s medium (DMEM), fetal bovine serum (FBS), calf bovine serum (Calf), phosphate-buffered saline (PBS, pH 7.4) and other tissue culture reagents were purchased from PAA Laboratories GmbH (Pasching, Austria). Type II crude porcine pancreatic lipase, p-nitrophenyl palmitate (PNP), α-amylase from porcine pancreas Type VI-B, α-glucosidase from saccharomyces cerevisiae, ρ-nitrophenyl-α-D-glucopyranoside (ρNPG) and all other chemicals were bought from Sigma-Aldrich Co (St. Louis, MO, USA). Antibodies for target proteins (PPARγ, C/EBPα, SREBP-1c, FAS, ACC and β-actin) were acquired from Cell Signaling Technology (Beverly, MA, USA). For qRT-PCR test, the TRlzol Reagent was obtained from Invitrogen (Carlsbad, CA), PrimerScriptTM 1st Strand cDNA Synthesis Kit from TaKaRa Bio Inc. (Otsu, Japan) and SYBR Green from PhileKorea Technology (Daejeon, Korea).
Dried root barks of mulberry were purchased from Omni Herb Company and extracted with 70% ethanol (MRE) and hot water (MRW). The extracts were filtered and evaporated under reduced pressure using a vacuum rotary evaporator. The extracts were then freeze-dried and stored at -70°C until used. The extraction yield obtained from MRE and MRW were 16.71% and 17.23% (w/w), respectively.
The α-amylase inhibitory activities of samples (MRE and MRW) were measured according to the method described by Ali et al. (26) and their α-glucosidase inhibitory activities were determined based on the method of Kim et al. (27). Pancreatic lipase inhibitory activities were determined according to the method of Kim et al. (28). In this study, acarbose and orlistat were used as positive controls. The percent inhibition of digestive enzymes was calculated as follows: Inhibition (%)=[(ODcontrol-ODsample)/ODcontrol]×100. The IC50 values were calculate by linear regression analysis.
3T3-L1 cells were cultured in T-flask with Dulbecco’s modified Eagle’s medium (DMEM) containing 10% Calf and 10 μg/mL of penicillin/streptomycin at 37°C in 5% CO2 atmosphere. Then, cells were subcultured after cells grown to more than 80-90% confluence. Two days after confluence (day 0), cells were stimulated to differentiate with differentiation medium containing DMEM with 10% FBS, 0.5 mM 3-isobutyl-1-methyl-xanthine, 5 μg/mL insulin and 1 μM dexamethasone for 2 days (day 2). Cells were then maintained in DMEM supplemented with 10% FBS and 5 μg/mL insulin for another 2 days (day 4), followed by culturing with DMEM with 10% FBS for an additional 4 days (day 8). Test samples were added in medium at various concentrations throughout the whole culture period (day 0~8).
The cytotoxicity of the sample was measured using MTT (3-(4,5-dimethylthiazol-2-yl)-2,5-diphenyltetrazolium bromide) method (29). Briefly, the cells were seeded at 104cells/well onto flat bottomed 96-well culture plates and treated with test samples at various concentrations. After incubation for 48 hr, the cells were incubated with MTT solution (5 mg/mL) at 37°C for 3 hr. The MTT-containing media was removed and dimethyl sulfoxide was added to dissolve formazan precipitates, then the optical density was measured at 570 nm using a microplate reader (Molecular Devices, Sunnyvale, CA, USA).
Cells were washed three times with phosphate buffered saline (PBS) and fixed for 30 min with 4% formaldehyde. The fixed cells were washed three times with distilled water. Oil red O (0.5% in 60% isopropanol) was diluted with water (3:2), filtered through Whatman paper and incubated with the fixed cells for 1 hr at room temperature. Plates were rinsed three times with distilled water and the stained fat droplets in the adipocytes were visualized by inverted microscopy and photographed. Spectrophotometric analysis of the stain was performed by dissolving the stained lipid droplets with isopropanol and measuring at 520 nm.
Extracted and quantified proteins (30 μg) from 3T3-L1 cells were separated with 10% SDS-PAGE gels and transferred onto PVDF membranes. The membranes were blocked in 5% skim milk containing TBST (Tris-buffered saline/Tween) for 3 hr. Then, the membranes were probed overnight with primary antibodies (PPARγ, C/EBPα, SREBP-1c, FAS and ACC). After being washed with TBST buffer, membranes were incubated with secondary antibodies for 1 hr. The expression levels of PPARγ, C/EBPα, SREBP-1c, FAS and ACC were determined using Supersignal West Pico chemiluminescence detection reagents (Pierce Biotechnology, Rockford, IL) and Konica X-ray film (Konica Co., Tokyo, Japan). Incubation with monoclonal mouse β -actin antibody was performed for comparative control.
Confluent cultures of 3T3-L1 cells in 60 mm dish were induced as previously described. Total RNA was isolated using TRlzol Reagent (Invitrogen, Carlsbad, CA) according to the manufacturer’s protocol. After cDNA was prepared from isolated RNA by using PrimerScriptTM 1st Strand cDNA Synthesis Kit (TaKaRa), the cDNA was used as a template for real time-PCR with the illumine ECOTM qPCR system (PhileKorea Technology, Daejeon, Korea). The specific primers are given in Table 1. The mRNA level was normalized using β-actin as an internal control. Analysis was carried out in triplicates.
All values were expressed in terms of mean±standard deviation (SD) of at least three independent experiments, tested by analysis of variance (ANOVA) with Duncan’s multiple range tests. Differences were considered to be statistically significant when the p-value was less than 0.05.
Results and Discussion
α-amylase and α-glucosidase, the two important starchhydrolyzing enzymes, are essential during digestion of carbohydrate. Inhibitors of these enzymes can decrease carbohydrate digestion and increase overall carbohydrate digestion time, causing a reduction in the rate of glucose absorption and consequently blunting the postprandial plasma glucose rise (30,31). In addition, inhibition of these enzymes may reduce excessive food calorie intake and retard fat accumulation. Therefore, development of α-amylase and α -glucosidase inhibitors may be one of the most effective approaches to controlling obesity and T2DM. As shown in Table 2, MRE showed dose-dependent inhibition on α -amylase activity with IC50 value of 7.86±0.36 mg/mL. MRW also exhibited some α-amylase inhibitory activity with IC50 value of 20.10±0.86 mg/mL. Acarbose was used as a positive control in this study, with IC50 value of 0.09 mg/mL. In addition, both MRE and MRW had appreciable inhibitory activity against α-glucosidase with IC50 values of 0.12±0.03 mg/mL and 1.15±0.02 mg/mL, respectively (Table 3). Especially, MRE inhibited α-glucosidase activity much stronger than positive control acarbose (IC50 value was 0.49±0.05 mg/mL). These results indicate that mulberry root bark extracts could be utilized as natural sources for potential anti-obesity and anti-diabetic application through inhibition of α-amylase and α-glucosidase activities.
Pancreatic lipase, also known as pancreatic triacylglycerol lipase, is very important in hydrolysis of triacyglycerols (32). Pancreatic lipase inhibition is a valuable pathway to retard fat absorption and therefore attenuates obesity (33). The pancreatic lipase inhibitory activity of ethanol and water extracts from mulberry root bark is shown in Table 4. MRE exhibited pancreatic lipase inhibitory activity, with IC50 value of 7.93±0.11 mg/mL. And the IC50 value of MRW was 75.13±0.81 mg/mL. The reference compound of orlistat was used in this study, with IC50 value of 0.01 mg/mL. Several studies also suggest that the extract of plants such as tea, soybean and ginseng inhibit pancreatic lipase activity (31,33). These results demonstrate that mulberry root bark has potential as a pancreatic lipase inhibitor.
Many current understandings of adipogenesis are based on 3T3-L1 cells. 3T3-L1 preadipocyte cells have been served as a well-established in vitro model to search for new health benefit food or agents for the obesity and related metabolic disorders in numerous studies (34). In this study, first of all, the effects of mulberry root bark extracts (MRE and MRW) on cell viability and the differentiation of 3T3-L1 cells were evaluated by MTT assay and Oil Red O staining assay. As evidenced by the MTT assay, no significant cytotoxicity was observed at concentrations up to 400 μg/mL, as compared with the non-treated control (Fig. 1A). Treatment of 3T3-L1 cells with MRE decreased adipocyte differentiation, in a dose-dependent manner, as indicated by a decrease in Oil Red O incorporation (Fig. 1C). The lipid accumulations were significantly decreased by 12.52% and 22.41% (p<0.05) at 100 μg/mL and 200 μg/mL of MRE, respectively (Fig. 1B). However, the inhibition of relative fat accumulation was not detectable in MRW. Many plant extracts and their derivatives showed the potent inhibitory activities on adipocyte differentiation and have been used as anti-obesity dietary supplements (35). These results indicate that mulberry root bark extract can efficiently block adipocyte differentiation and may have a potential benefit in preventing obesity.
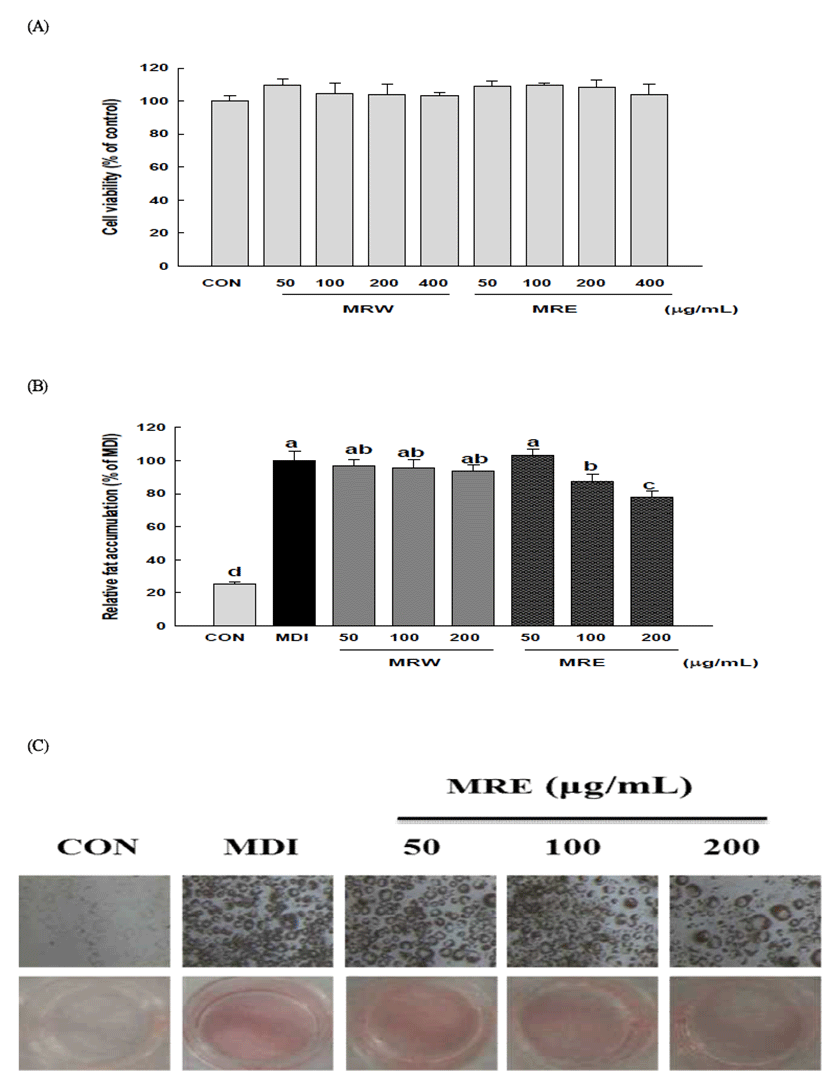
To gain a better understanding of the molecular mechanisms underlying the anti-adipogenic effect, we conducted qRT-PCR and Western blotting analysis to examine the effect of MRE on the expression of key transcriptional factors. PPARγ and C/EBPα are necessary and should be sufficient for adipogenesis in vivo and in vitro (10,11). We observed that PPARγ and C/EBPα were strongly inhibited by MRE at the transcriptional levels (Fig. 2). The mRNA level of PPARγ was reduced up to 70.97% and that of C/EBPα mRNA was decreased by up to 55.09%, respectively. Consistent with the mRNA expression, the protein expression levels of transcription factors (PPARγ and C/EBPα ) showed a similar alteration pattern (p<0.05, Fig. 3). We also determined the gene expression of SREBP-1c, a key transcription regulator which is well-known upstream regulators of PPARγ in the adipogenesis pathway (12). It showed that the SREBP-1c mRNA was suppressed significantly by MRE (Fig. 2). Furthermore, treatment of MRE induced down-regulation of SREBP-1c protein expression (p<0.05, Fig. 3). These results indicate that MRE suppresses SREBP-1c as the upstream regulator of PPARγ at the transcriptional level. However, the deep investigation of molecular mechanism of MRE on PPARγ upstream signaling pathway need to be studied further. Consequently, these observations suggest that MRE inhibits aipogenesis during adipocyte differentiation.
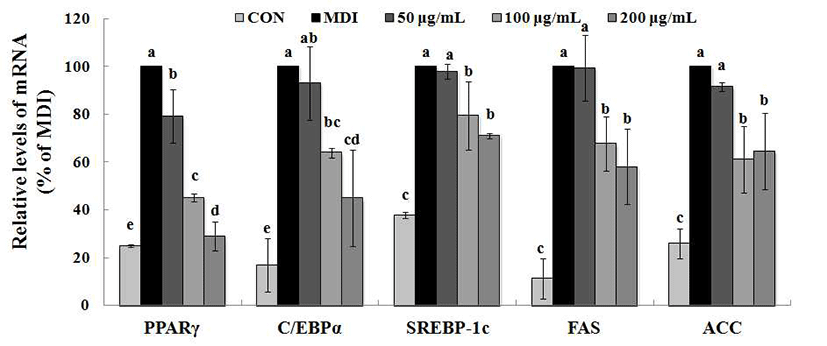
FAS and ACC are the two key enzymes in lipogenesis, a fact that renders they are important targets of anti-obesity research (36). Therefore, we further studied whether MRE regulated the genes expression of FAS and ACC. Treatment with MRE inhibited the transcription of FAS in a dose-dependent manner (p<0.05, Fig. 2). It also induced down-regulation of ACC transcription compared with fully differentiated adipocytes. Moreover, the protein level of FAS was fallen dramatically up to 97.14% (p<0.05, Fig. 3). ACC protein expression was decreased by up to 57.08% upon MRE addition (p<0.05, Fig. 3). These results indicate that MRE inhibits lipogenesis during adipocyte differentiation.
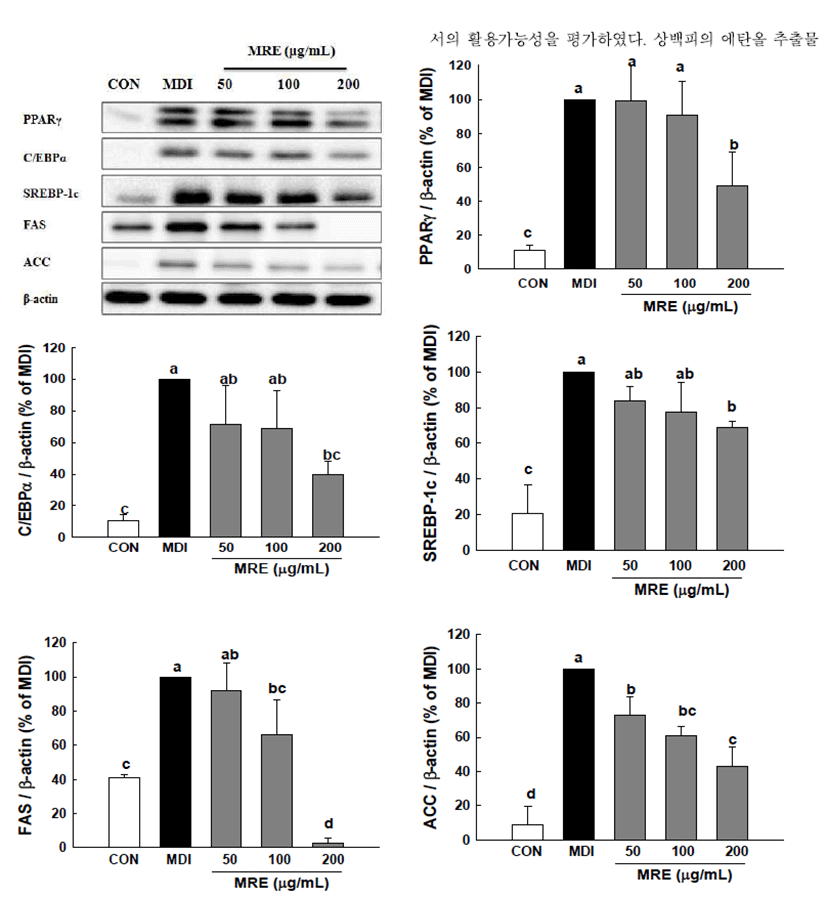
Conclusion
Our investigations indicate that mulberry root bark is an effective inhibitor of α-amylase, α-glucosidase and pancreatic lipase. In addition, MRE has potential of inhibiting adipogenic differentiation in 3T3-L1 cells. These effects of MRE may work on multiple molecular targets and complex mechanisms modulating the expression of transcription factors such as PPARγ, C/EBPα and SREBP-1c, and suppressing the expression of lipogenesis related proteins such as FAS and ACC. Consequently, based on these findings, we suggest that mulberry root bark can be utilized as a functional resource having pharmacological and health-promoting effects for anti-obesity. Additional studies of active compounds responsible for the anti-obese effect of MRE are currently underway.
요 약
본 연구에서는 상백피의 소화효소 저해활성과 3T3-L1 전지방세포의 분화 억제능을 기반으로 항비만 효능소재로 (MRE)은 α-amylase와 α-glucosidase, pancreatic lipase를 활 용한 소화효소 저해활성 평가 실험에서 각각 7.86±0.36, 0.12±0.03, 7.93±0.11 mg/mL 의 IC50 값을 보이며 우수한 억제 활성을 나타냈다. 또한 3T3-L1 전지방세포를 활용한 세포분화억제효능실험에서 MRE 처리군의 세포내 지방 축 적율은 농도 의존적으로 감소되었다. 상백피의 항비만 작 용 기전을 구명하기 위하여 adipogenesis 및 lipogenesis와 관련된 유전자 발현양상을 분석한 결과, 상백피 추출물 처 리군에서는 생체내 지방대사 조절에 중요한 역할을 하는 FAS와 ACC 뿐 아니라 adipogenesis와 lipogenesis와 관련된 주요 전사요소인 PPARγ와 C/EBPα, SREBP-1c의 유전자 발현이 현저하게 억제되었다. qRT-PCR 분석 결과, 상백피 추출물의 anti-adipogenesis 효능은 전사단계에서의 관련 유 전자 발현억제에 기인한다고 판단되었다. 본 실험결과 상 백피 추출물은 전지방세포의 분화와 세포내 지질합성을 저해하고 비만과 관련 된 소화효소에 대한 저해활성을 나타 내었다. 이러한 결과를 기반으로 상백피의 비만 예방 소재 로서의 잠재적인 가능성을 확인하였다.