Introduction
Raspberries, which belong to the family Rosaceae, have a high economic value as fruiting species and are widely distributed in Southeast Asian countries (1). Recent studies (2-4) have found that raspberries contain an abundant amount of polyphenols (278 to 714 mg gallic acid equivalents/100 g), anthocyanins (325 mg cyanidin 3-glucoside equivalents /100 g), and vitamin C (9.2 mg/100 g). Further, daily raspberry intake has been shown to confer protective effects against several degenerative diseases (5). Unfortunately, raspberries are highly perishable as they readily rot when infected by gray mold (Botrytis cinerea), resulting in a loss of firmness and a darkening of their attractive red color (6). In order to retain fruit quality during long-term storage, most raspberries are currently preserved in frozen form after harvest. However, frozen raspberries result in a significant dripping loss once they are thawed, and the inevitable loss of flavors and nutritional value (7). Therefore, other processing techniques are required for developing nutritional raspberry products.
Drying is the most common and effective method for food preservation and shelf-life extension (8). The drying process leads to a reduction of the moisture content to a satisfactory level, allowing safe storage over an extended period. In addition, for small fruits like raspberries and mulberries, it also results in a substantial water loss, increasing sweetness and flavor. Compared to other processed raspberry products such as juice, candy, and syrup, dried raspberries do not contain any harmful additives (such as antiseptics, colorants, or aromatic chemicals), making them more natural, healthier, and popular with consumers. Solar drying is a widely used process to preserve foods, but problems associated with solar drying are well documented (9). Alternatively, dehydration in air driers at controlled temperatures and air flow ensures the establishment of the appropriate moisture level and better preservation of product quality. At present, dehydration using hot-air (60~100°C) is common for various fruits and vegetables (10,11). However, the product quality and flavor resulting from hot-air drying are often reduced due to excessive temperature and drying time. More importantly, reduction of the moisture content to a low level can result in food that is too difficult to eat for some consumers, such as children and the elderly. Therefore, researchers are currently focused on developing semi-dried foods such as spices (pepper), fruits (persimmons and tomatoes), and meats (pork and beef) with softer tastes, retained nutrient values and antioxidant activities, as well as extended shelf-lifes (12-15). Increasing demand for high quality and nonperishable dried fruit requires the optimization of the dehydration process, especially with regards to drying temperature and processing time. Such optimization may critically affect not only the efficiency of the drying process, but also the quality of the final dehydrated product (16).
In pursuit of this optimization, this study applied hot-air drying (60~100°C) and cool-air drying (20~40°C) under vacuum to produce semi-dried raspberries. The aim of the present research was to investigate the influence of drying temperature and processing time on the quality of semi-dried raspberries, and to predict optimal drying conditions so as to minimize nutrient losses during the drying process by developing statistical models according to response surface methodology (RSM). The total loss of vitamin C (VC), total phenolic content (TPC), and ellagic acid (EA) were used to evaluate the quality of the final raspberry product.
Material and methods
Raspberries (Rubus coreanus Miq.) were obtained from the city of Mungyeong in the Gyeongbuk area of Korea, and were harvested by hand and kept refrigerated at 4°C before drying. The moisture content of the fresh and dried mulberry fruits were determined by AOAC method no. 934.06 (AOAC, 1990).
Hot-air drying was conducted in a lab-scale convective air dryer (0~2 m/s, 10~120°C). The temperature was set constant between 60 and 100°C using a temperature controller. Cool-air drying was carried out in a forced circulation and batch type (Shinil, Seoul, Korea). This device allowed for temperature ranges between 20 and 40°C, with 1.0°C variation. The air velocity was fixed at 1 m/s. For both drying processes, approximately 100 g of the fresh harvested raspberries were weighed, put on an aluminum plate, and placed in the respective drying devices. After drying, the moisture residue content was recorded and the dried raspberries were preserved in a zipper bag at -20°C until further extraction and chemical analysis.
A 5 g of semi-dried raspberries were collected in a centrifuge tube (50 mL) and macerated with 25 mL acidified methanol (0.1% HCl) for 10 min. The macerated raspberries were then homogenized using a Polytron PT1200 homogenizer (Kinematika, Littau, Switzerland) and ultrasonicated for 30 min in a laboratory ultrasonic bath (JAC Ultrasonic 2010P, Jinwoo Engineering Co., Ltd., Hwasung, Korea). Afterwards, the treated homogenate was centrifuged at 2,000×g for 20 min at 4°C and the supernatant was collected in a brown vial. A 2 mL extract was injected through a 0.45 μm PTFE syringe filter for analysis of TPC, VC, and EA content.
The TPC was determined using the Folin-Denis method described by Singleton et al. (17) with modifications. A 100 μL of previously filtered raspberry supernatant was mixed with 50 μL of Folin-Ciocalteu reagent and 300 μL of 2% Na2CO3. After keeping at room temperature for 15 min, 1 mL of distilled water was added and the absorbance was measured at 725 nm. The results were expressed as a percentage (%) of loss ratio as compared to the untreated raspberry sample (dry basis).
Vitamin C content was analyzed using HPLC (JASCO International Co., Tokyo, Japan) with an XTerra C18 reversed-phase column (250 mm×4.6 mm, Waters, USA), according to the method described by Phillips et al. (18) with minor modifications. The mobile phase consisted of 0.15 % aqueous formic acid. A 60 μL of previously filtered raspberry supernatant was injected into the HPLC system and eluted under isocratic conditions at 1 mL/min. Absorption was detected at 255 nm with a UV detector. Ascorbic acid (10~30 μg/mL) was used as an external standard and Fig. 1(a) shows the peak of ascorbic acid eluted at 6.8 min. Under these conditions, vitamin C content in dried raspberries got fully separated as shown in Fig.1(b). The results were expressed as a percentage (%) of loss ratio as compared to the untreated raspberry sample (dry basis).
Ellagic acid was separated and identified using HPLC (JASCO International Co., Tokyo, Japan) with a UV detector.
Separation was performed on an XTerra C18 reverse phase column (250 mm×4.6 mm, Waters, USA) at 30°C. The mobile phase consisted of 5% aqueous acetic acid (in water) and 0.1% formic acid (in acetonitrile solution). A 60 μL of the previously filtered raspberry supernatant was injected, and a flow rate of 0.8 mL/min was used. Absorption was detected at 280 nm and retention time was 60 min. Ellagic acid ((10-50 μg/mL) was employed as an external standard and eluted at 24.8 min as shown in Fig. 2(a). Under these conditions, individual peaks shown in Fig. 2(b) suggested a good separation of chemical compounds in dried raspberries. The results were expressed as a percentage (%) of loss ratio as compared to the untreated raspberry sample (dry basis).
A central composite design (CCD) generated by SAS software (9.3, SAS Institute, Cary, NC, USA) was employed for the optimization of the drying process. The drying temperature and processing time for the hot-air drying process were 60~100°C and 2~10 hr, respectively. For the cool-air drying process, these values were 20~40°C and 22~66 hr, respectively. Experimental data were fitted into an empirical second order polynomial model using regression analysis and presented in the following equation:
In the equation above, Y represents the independent responses, ß0, ßi, ßii, and ßij represent the regression coefficients of the process variables for the intercept, linear, quadratic, and cross product terms, respectively, and ε represents the error. Statistical significance of the coefficients in the regression equation was checked by analysis of variance (ANOVA). The fit of the polynomial model equation to the responses was evaluated using both the R-squared (R2) coefficient and the F-test.
Statistical analyses, including analysis of variance (ANOVA), fit statistics, and canonical analysis, were completed using SAS software (9.3, SAS Institute, Cary, NC, USA). Three-dimensional response surface plots and overlapped contour plots were generated using STATISTICA 8.0 (Statsoft Inc., NY, USA).
Results and discussion
When analyzing food, ascorbic acid is usually taken as an important index of nutrient quality. It is a labile compound that may lose activity due to several factors, including pH, moisture content, oxygen, temperature, and metal ion catalysis (19,20). According to Puupponen-Pimiä et al. (21), raspberries contain relatively high amounts of bioactive compounds (flavonoids, phenolic acids, and tannins). Ellagic acid is the main phenolic compound in both fresh and dried raspberries, forming 77-88% of the phenolic compounds analyzed, which is affected by many different factors during food processing and storage, including light, temperature, and humidity (22-24).
In this study, conventional hot-air and cool-air were employed for producing semi-dried raspberries. The effects of temperature and processing time on moisture residue ratio (MRR), vitamin C loss ratio (VCLR), total phenolic content loss ratio (TPCLR), and ellagic acid loss ratio (EALR) were investigated. RSM was used in order to optimize the drying processes so as to minimize the losses of functional compounds and to improve fruit quality.
Table 1 shows that the highest MRR (80.22%) for hot-air drying was obtained at 60°C for 6 hr, while the lowest MRR (25.29%) was observed at 80°C for 10 hr. Table 1 also indicates that the highest temperature (100°C) used in this test led to the highest TPLCR (47.18%) and VCLR (90.89%). However, the highest EALR (61.63%) was observed in test 8 at 80°C, using the longest processing time (10 hr) in the CCD matrices.
ANOVA results in Table 2 suggest that hot-air fitted models for MRR, TPCLR, VCLR, and EALR were significant (p<0.05), with R2 values of 0.9222, 0.8557, 0.9508, and 0.7912, respectively, indicate good fitness of the selected second order polynomial model to the data. Table 2 also reveals that the process variables of both drying temperature and processing time proved significant (p<0.05) on MRR, TPCLR, and VCLR; however, EALR was only significantly influenced by processing time. Stationary points for the fitted models for MRR and EALR were saddle points, while stationary points for TPCLR and VCLR were maxima (Table 2). Fitted models for MRR, TPCLR, VCLR, and EALR are presented below :
Based on the fitted models, three-dimensional surface plots for MRR, TPCLR, VCLR, and EALR under hot-air drying conditions were constructed. Fig. 3(a) shows that MRR is linearly dependent on both processing time and drying temperature, decreasing from 100 to 0%. Surface plots for VCLR and TPCLR in Fig. 3(b) and (c) show good quadratic effects, and with the increase of processing time and drying temperature, VCLR and TPCLR increase. Fig. 3(b) demonstrates that high temperature treatment leads to tremendous decrease (up to 80%) in ascorbic acid content, and similar results were reported in previously studies (25-27). Gregory (19) has attempted to provide a rational explanation, proposing that the substantial loss of ascorbic acid during hot-air treatment is induced by the heat-mediated oxidation of ascorbic acid to dehydroascorbic acid, followed by hydrolysis to 2,3-diketogulonic acid and further polymerization to form nutritionally inactive products. However, unlike VCLR, TPCLR is limited to 40%, as shown in Fig. 3(c). Dewanto et al. (28) reported that TPC does not change significantly during thermal processing of fruits, and rather, thermal processing could enhance nutritional values by releasing bio-accessible compounds. Chism and Haard (29) assumed that TPC of fruits and vegetables is usually primarily contained in the vacuoles, and that thermal processes might accelerate the release of phenolic compounds as a result of the breakdown of cellular constituents. This explanation might account for the limited loss of phenolic acids under hot-air drying conditions. In addition, it is also noticed that EALR increases linearly with heating temperature and processing time, reaching over 60%. Judging from the angle of the surface plot in Fig. 3(d), and processing time affects EALR more significantly.
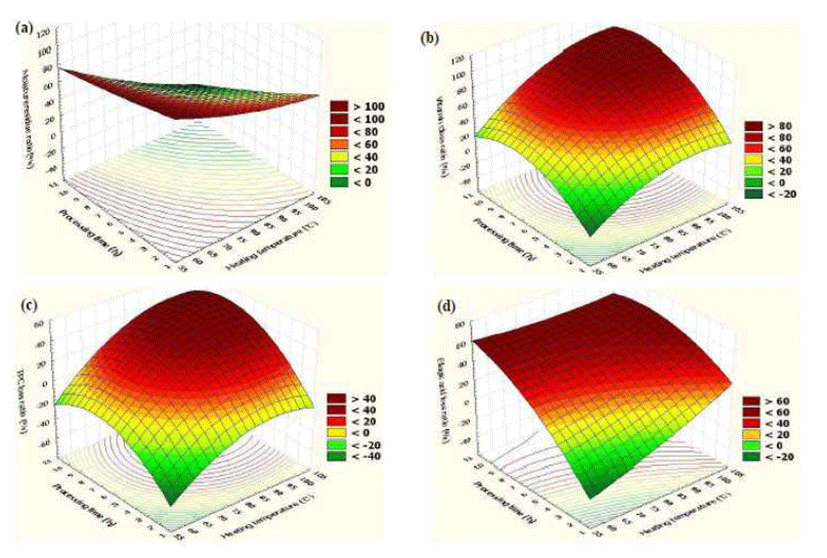
The results for MRR, TPCLR, VCLR, and EALR for cool-air drying are presented in Table 3. The temperature for cool-air drying ranged from 20 to 40°C, and while processing time ranged from 22 to 66 hr. Raspberry samples processed at 20°C for 22 hr received the highest MRR (86.52%), and the lowest TPCLR (17.16%), VCLR (27.36%), and EALR (32.63%). The highest TPCLR (70.73%) and the lowest MRR (36.83%) were detected in test 4, at 40°C for 6 hr, and the highest VCLR (60.54%) and EALR were found in test 8, at 30°C for 66 hr, which was the longest time tested for cool-air drying.
ANOVA results in Table 4 show that the fitted models for MRR and TPCLR are highly significant (p<0.001), while high R2 values of 0.9796 and 0.9882, respectively, indicate a good fit to the data and reliability for further prediction. VCLR and EALR models are also highly significant (p<0.05), with R2 values of 0.9141 and 0.8119, respectively, indicating a good fit. The fitted models for cool-air drying are presented as follows :
Three-dimensional surface plots for MRR, TPCLR, VCLR, and EALR for cool-air drying are shown in Fig. 4(a)~(d). Fig. 4(a) exhibits a good quadratic effect, and drying temperature and processing time show negative effects on MRR. MRR is reduced from 80% to 40% when the drying temperature is increased from 20 to 40°C and drying time is extended to 70 hr. According to ANOVA results in Table 4, processing time is the only variable significantly affecting ascorbic acid reduction during the cool-air drying process, which is also demonstrated in Fig. 4(b) Fig. 4(c) and (d) show that TPCLR and EALR are high (more than 70% as predicted) when cool-air treatment is used. Recent studies (30,31) suggest that this might be due to the hydrolysis, oxidation, and polymerization of phenolic compounds. It is also noteworthy that TPC loss was higher in cool-air dried raspberries than in hot-air dried ones, because low temperature treatment tends to preserve thermally sensitive compounds from decomposition in most circumstances. But Ding et al. (32) and Trejo-Gonzalez et al. (33) both referred a possible explanation that the temperature activated enzyme polyphenoloxidase (PPO) can induce oxidation and degradation of phenolic compounds. According to Concellón et al. (34) the optimal temperature for fruit PPO activity is in the range of 30-40°C, which explains why cool-air drying under 40°C causes higher TPCLR in raspberry fruits than hot-air drying.
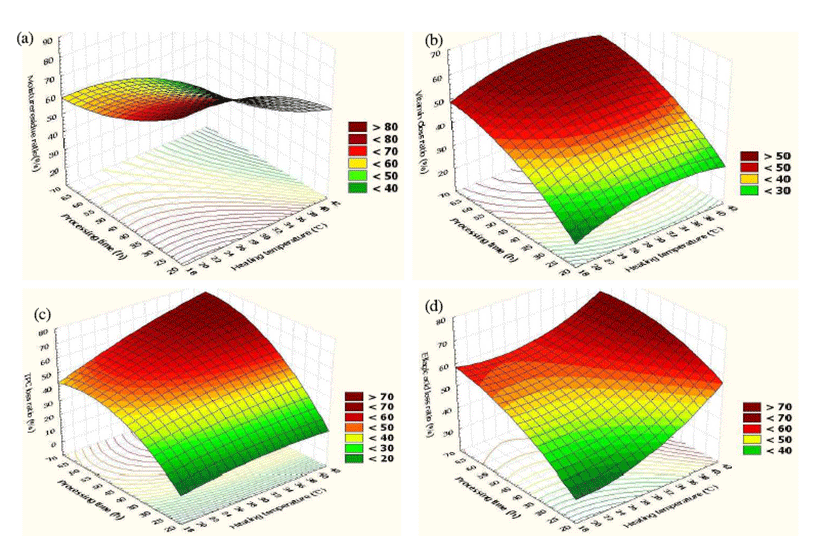
Optimization is an important method in the food industry for monitoring critical process variables and yielding high-quality products. Madamba et al. (35) reported that the application of optimization to drying processes could yield mathematical models, which could help minimize nutrient loss, a factor that directly correlates to the quality of food products. The main goal of this research was to semi-dry raspberries and to determine whether the best processing conditions are found through traditional hot-air processing or through the use of cool-air. Since only two process variables were involved in this study, the most effective approach could be the superimposed contour diagrams of different response variables. Fig. 5(a) and (b) show the superimposed contour plots for MRR, TPCLR, VCLR, and EALR, as affected by both drying temperature and processing time under hot-air and cool-air, respectively. The optimum conditions for drying of raspberry fruits were set at 45% MRR, with minimal values for TPCLR, VCLR, and EALR. With these parameters set, the optimal conditions for semi-drying raspberries with hot-air were found to be a drying temperature of 65.8°C and a processing time of 4.3 hr, at which VCLR, TPCLR, and EALR were predicted to be 51.3%, 2.8%, and 48.8%, respectively. Meanwhile, the optimal conditions for cool-air drying were calculated to be 21.3°C and 28.2 hr, with VCLR, TPCLR, and EALR values of 21.7%, 17.2%, and 15.9%, respectively.
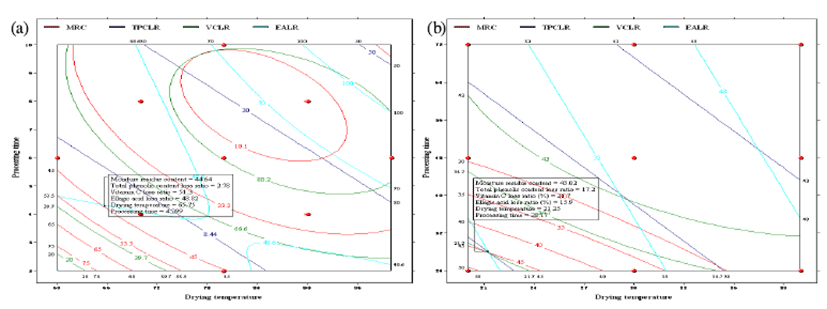
요 약
ANOVA 분석을 통해 건조온도와 건조시간이 열풍건조 와 냉풍건조의 중요변수임을 알 수 있었으며 복분자의 반건 조를 위해서는 열풍건조의 경우 65.8°C, 4.3시간이 적당하 였고 냉풍건조의 경우 비슷한 정도의 건조수분함량까지 28.2시간이 걸렸다. 그러나 건조온도가 높아지면 vitamin C와 ellagic acid의 함량이 줄어드는 등 품질적 열화가 심하 게 나타났다. 또한 생리활성물질들은 냉풍건조에서 전반적 으로 더 잘 보존 되었으며 총폴리페놀의 함량에서는 더욱 그러하였다. 따라서 냉풍건조방법은 고품질의 반건조 복분 자 제조에 적합한 건조방법이며 최적 건조조건은 21.3°C, 28.2시간으로 나타났다.