1. Introduction
During the rapid industrialization period, the widespread use of persistent organic pollutants (POPs) began. These synthetic chemicals were commercially introduced because of their economic benefits as they were cheaper than natural substances (Duttagupta et al., 2020). However, products discarded in landfills and waste sites after use persist for decades and continuously release breakdown products into the surrounding terrestrial and aquatic ecosystems (Frederic et al., 2018). POPs are globally concerning chemicals because they can undergo both bioaccumulation and biomagnification within ecosystems, causing significant negative impacts due to their toxicity (Ochs et al., 2024). The biomagnification of POPs throughout the food chain can result in higher toxic effects on animals at higher trophic levels (Hoondert et al., 2020). Exposure to POPs is associated with various ecotoxicological outcomes, including immunotoxicity, dermal effects, congenital disabilities, cancer, reproductive impairment, and overall population decline. Numerous wildlife species suffer from immunodeficiency syndromes due to specific POPs, such as polychlorinated biphenyls (PCBs), chlordane, hexachlorobenzene (HCB), dioxins, toxaphene, and dichlorodiphenyltrichloroethane (DDT) (Lee et al., 1998). POPs have been managed globally following the adoption of the Stockholm Convention. Risk assessment, which is essential for the safe management of these toxic substances, is the process of scientifically analyzing and evaluating the potential impacts of chemicals or environmental pollutants on human health and the environment. Research data on the toxicity, safe exposure standards, and monitoring data of the
relevant substances are essential components of any risk assessment. Although extensive research has been conducted on pollutants listed as POPs after the Stockholm Convention, some persistent, bioaccumulative, and toxic (PBT) substances still remain in the environment and require further study. Among them, pentachlorothiophenol (PCTP) is restricted in use in the United States and is treated as both a PBT and a very persistent, very bioaccumulative (vPvB) substance in Europe, further limiting its use. However, compared to other POPs and PBT substances, toxicity data and research on PCTP are both lacking. PCTP has been widely used in various industrial applications, including mercaptide reactions for the production of rubber and other chemicals (KULKA, 1959). Despite its industrial utility, PCTP is known as a limiting substance due to its chemical stability and resistance to environmental destruction. According to an evaluation by the European Chemicals Agency (ECHA) under EU chemicals legislation, PCTP meets the criteria for PBT/vPvB substances.
Another well-known PBT substance, HCB, shares similar concerns and usage history. Previously used as a fungicide for seed treatment and in other industrial applications like fireworks and aluminum manufacturing, the production and use of HCBs have been largely banned under the Stockholm Convention due to their toxic effects and environmental persistence. However, HCB still appears as a byproduct in the manufacturing and incineration processes of other chemicals.
Because PCTP and HCB are structurally similar and share industrial origins, investigating the toxicity profile, analytical detection methods, and environmental monitoring studies of HCB should provide valuable insights for the risk assessment of PCTP. The extensive documentation of HCB as a harmful compound can serve as a fundamental reference to help understand and predict the behavior and impact of PCTP on the environment and human health.
This review integrates the existing literature on the toxicity, analytical methodologies, and monitoring data for PCTP with relevant findings for HCB. By incorporating the well-established knowledge base for HCB, this review will highlight the potential risks posed by PCTP and emphasize the need for rigorous environmental and health safety assessments.
2. Toxicity
According to the Hazardous Substances Data Bank (HSDB) of the U.S. National Library of Medicine (NLM), no skin irritation was observed in humans after 24-hour exposure to PCTP, although minor irritation to the eyes and respiratory system was noted. However, studies on the toxicity of PCTP in humans remain quite limited. While there are some studies on animal toxicity and ecotoxicity, they are somewhat outdated and appear to require revision. Various qualitative toxicity experiment data were identified in the HSDB of the NLM, including two sets of qualitative toxicity data observed in rats, rabbits, guinea pigs, and cats. In the first study, rats and rabbits were exposed to 1 mg/L of PCTP dust for 1 hour, resulting in slight respiratory irritation, with rabbits showing greater sensitivity than rats. In the second study, rats, guinea pigs, rabbits, and cats were exposed to 2.050 g of evaporated PCTP for 4 hours. All animals except rats exhibited mucous membrane irritation, and conjunctivitis symptoms persisted in cats and rabbits the following day. For skin exposure, PCTP soaked in water and oil was applied to the ears of rabbits using a swab and monitored for 24 hours. This resulted in mild inflammation, erythema, and light scab formation, but symptoms resolved within a week. According to the European Commission/European Chemical Substances Information System (ESIS); IUCLID Dataset, when 500 mg of PCTP was exposed to the eyes of rabbits for 24 hours, mild irritation was observed.
Quantitative acute toxicity data were evaluated in rats and mice using data obtained from the International Uniform ChemicaL Information Database (IUCLID) dataset provided by the European Commission/European Chemical Substances Information System (ESIS). Oral and intraperitoneal administration of PCTP revealed different median lethal dose (LD50) values for each species. For oral administration, rats showed higher LD50 values compared to mice, indicating lower toxicity. However, mice were highly sensitive to intraperitoneal administration, with LD50 values ranging from 100-200 mg/kg to 280 mg/kg. These results suggest varying toxic mechanisms depending on the route of exposure, necessitating further research for the safe handling of PCTP. Additionally, PCTP exhibits bioaccumulative properties, implying potential accumulation in aquatic organisms and the environment. Therefore, assessing aquatic toxicity is crucial. In static bioassays, the absolute lethal concentration (LC100) values for Brachydanio rerio (zebrafish) and Leuciscus idus (golden orfe) were determined. For L. idus, the test substance, composed of 88% PCTP, 2% tetrachlorodithiol, and a total of 10% pentachlorobenzene disulfide and pentachlorophenol combined, had an LC100 value of 1.0 mg/L. For B. rerio, an LC100 value of 2.8 mg/L over 96 hours was observed. These toxicity data are essential for developing safety guidelines for PCTP use.
The HSDB further notes that injecting PCTP into chicken embryos resulted in a 4.5-fold increase in hepatic protoporphyrin levels, indicating biochemical changes in the liver. In chick embryonic liver cells, HCB metabolism led to porphyrin accumulation within 24 hours, with the pattern of accumulation resembling that observed in oral administration studies in other experimental animals (Debets et al., 1981). Additionally, PCTP as a metabolite of HCB, acts as an uncoupler of oxidative phosphorylation in the mitochondria of rat livers, affecting cellular energy production (Smejtek et al., 1983). The addition of the monooxygenase inhibitor piperonyl butoxide or ascorbic acid reduced the irreversible binding of these metabolites (Debets et al., 1981). Studies on the inhibitory effects of HCB and its metabolites on porphyrinogen-carboxy-lyase (PCL) activity demonstrated that various compounds, including HCB, pentachlorobenzene, tetrachlorobenzene, and PCTP, inhibited PCL activity, suggesting that sulfur-containing metabolites induce porphyrin accumulation. Moreover, tetrachlorohydroquinone and trichlorophenol exhibited the most substantial inhibitory effects on PCL activity (de Calmanovici et al., 1986). Toxicity studies for PCTP above are presented in Table 1.
Subjects | Toxic effects | References |
---|---|---|
Human | Eye and respiratory irritation | ESIS (2000) |
Rats and rabbits | Respiratory irritation | ESIS (2000) |
Rats, rabbits, guinea pigs, and cats | Mucous membrane irritation, conjunctivitis | ESIS (2000) |
Rabbits | Mild inflammation, erythema | ESIS (2000) |
Mice | Oral administration LD50 | ESIS (2000) |
Rat mice | Intraperitoneal injection LD50 | ESIS (2000) |
Danio rerio (zebrafish) | LC100 | IUCLID (2009) |
Leuciscus idus (golden orfe) | LC100 | IUCLID (2009) |
Chicken embryos | Increase in hepatic protoporphyrin levels | ESIS (2000) |
Rat liver mitochondria | Uncoupler of oxidative phosphorylation in the mitochondria of rat livers | Smejtek et al. (1983) |
Chick embryo | porphyrin accumulation in liver cells | Debets et al. (1981) |
Female chbbthom rats | Inhibitory effects on porpyrinogen-carboxy-lyase (PCL) activity | de Calmanovici et al. (1986) |
PCTP is generated as a significant metabolic intermediate during the breakdown of HCB and pentachloronitrobenzene (PCNB) (Koss et al., 1978). and has been assessed for its toxic effects as a primary metabolite of HCB and PCNB in various animal models (To-Figueras et al., 1997). The presence of PCTP and pentachloroanisole in the livers of rats treated with HCB confirms that PCTP is a metabolite of HCB and PCNB (Koss et al., 1976; Koss et al., 1979). Additionally, metabolites including PCTP have been detected in the excreta of various animal species (rats, fish, and quail) following HCB administration (Böger et al., 1979). Investigations of metabolites formed after administering PCNB to rats also confirmed PCTP as a metabolite (Renner, 1980).
In a study where F344 rats were administered HCB every other day for 103 days, pentachlorophenol, 2,3,5,6-tetrachlorobenzene-1,4-diol, and PCTP were identified as metabolites, with female rats exhibiting higher excretion levels (Rizzardinie and smith, 1982). Oral administration of HCB (178 μmol/kg, 50 mg/kg) to rats resulted in the detection of approximately 0.1 nmol of PCTP in the liver, confirming its formation during HCB metabolism (Clayton and Clayton, 1981-1982). Following oral administration of 770 mg/kg HCB for one month, PCTP was detected in all organs, with higher concentrations in the liver of female rats compared to males. Moreover, PCTP and HCB levels in females did not significantly decrease over seven weeks and declined more slowly than in males (Richter et al., 1981). Pentachlorophenyl-mercapturic acid was detected and isolated from the urine of rats treated with HCB, providing evidence for the incorporation of sulfur via the reaction of HCB with glutathione (GSH) (Testa and Jenner, 1978). The protozoan Tetrahymena thermophila produces pentachlorothioanisole (PCTA) through a PCTP intermediate during metabolism of the fungicide PCNB. In this process, Tetrahymena cells absorb aromatic thiols like PCTP, methylate them, and then excrete methylated products (Drotar and Fall, 1986). PCTP was identified as a metabolite of PCNB in golden orfe fish (Neilson and Allard, 2007). In addition, research on the metabolic processes of HCB in aquatic environments has identified several metabolic products following exposure to both radiolabeled 14C-HCB and non-radioactive HCB in blue mussels (Mytilus edulis) (Bauer et al., 1989). Among the metabolites isolated were pentachlorothioanisole (PCTA), S-(pentachlorophenyl) thioglycolic acid (PCPSA), and 1,4-bis(methylthio)-2,3,5,6-tetrachlorobenzene (Bis-MTTCB). This highlights the complexity of HCB metabolism in aquatic organisms. PCNB (pentachloronitrobenzene) has three main metabolic mechanisms (Torres et al., 1996). The first is the reduction pathway, in which PCNB is reduced by microorganisms to PCA (pentachloroaniline) and TCA (tetrachloroaniline), thereby reducing the toxicity of PCNB. The second mechanism involves the formation of sulfur-containing metabolites, where PCNB reacts with glutathione to be converted into pentachlorothiophenol (PCTP) and pentachlorothionisol (PCTA). PCTP is formed through the substitution of nitro groups, while PCTA is produced during microbial metabolism. The third pathway is hydroxylation, in which PCNB is converted to pentachlorophenol (PCP) through a hydroxylation reaction that removes nitro groups. The resulting PCAN (pentachloroanisole) serves as a subsequent metabolite. Together, these three pathways create complex mechanisms through which PCNB interacts during degradation to produce a variety of metabolites.
The above studies confirm that PCTP is a major metabolite formed during the metabolism of HCB and PCNB, enhancing our understanding of the presence and toxic effects of these metabolites. These findings provide essential foundational data for protecting aquatic environments and ecosystems. The mechanism of PCTP production during the metabolic processes of HCB and PCNB is shown in Fig. 1 (Teng et al., 2017).
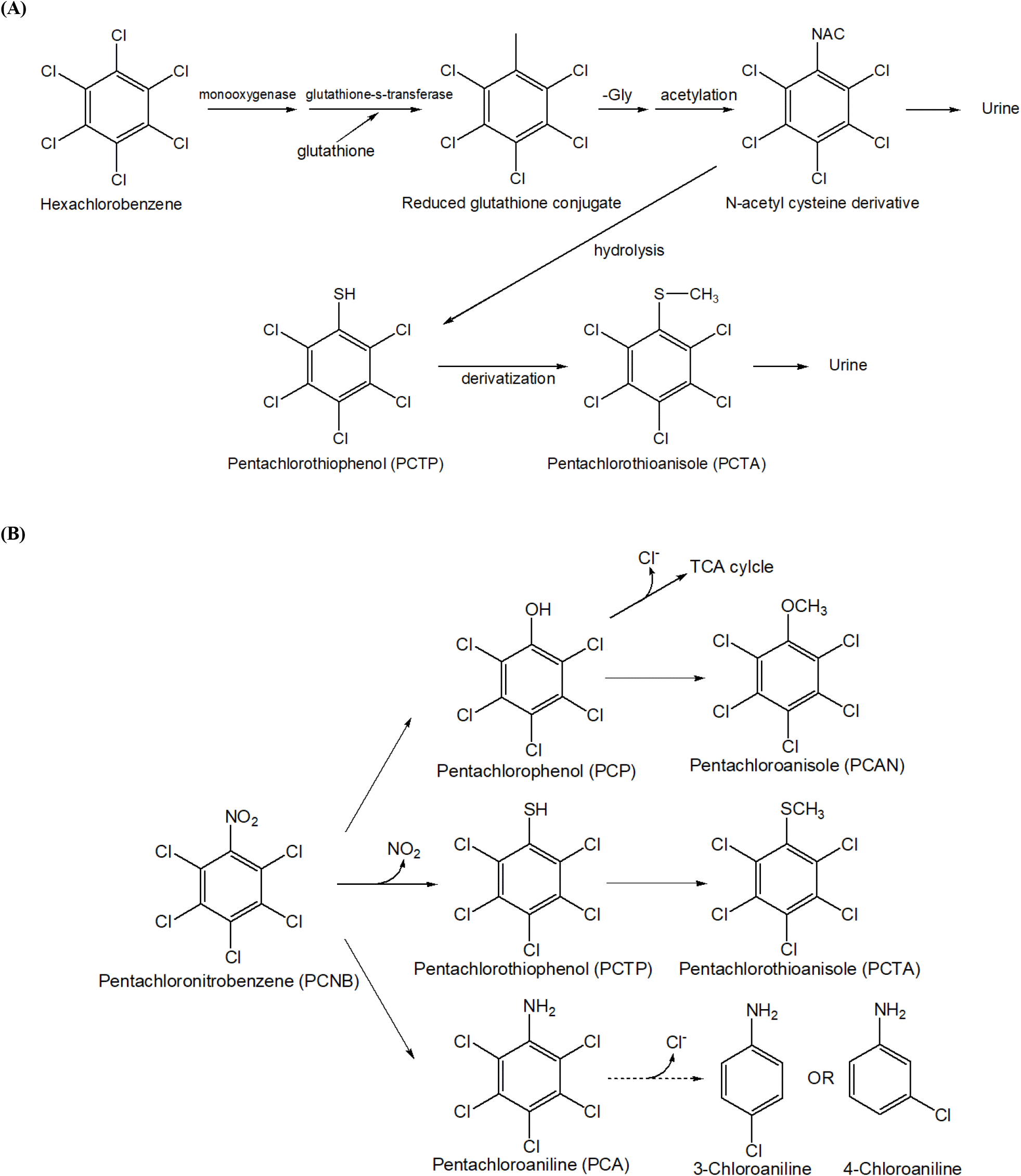
3. Analytical methods
To evaluate the exposure levels and risks of PCTP, extensive monitoring data is required. Generating such data necessitates the use of appropriate and validated analytical methods. However, few studies have been published on analytical methods for quantifying PCTP, and some methods have been developed as part of established protocols for detecting metabolites of substances like HCB.
The following method was developed to monitor HCB and its metabolites in human serum and feces. For fecal samples, approximately 250 mg was dried at 65°C, then digested with 2 N NaOH at 70°C for 4 hours. After extraction with benzene and subsequent gas chromatography analysis, HCB and its metabolites were detected. In urine samples, 4 mL is hydrolyzed with 2 N NaOH at 70°C for 3 hours, followed by benzene extraction and gas chromatography analysis for monitoring HCB and its metabolites (To-Figueras et al., 1997; To-Figueras et al., 2000).
After intraperitoneal injection of 14C-hexachlorobenzene into female Wistar rats, the levels of pentachlorophenol (PCP) and its metabolites tetrachlorohydroquinone and PCTP were measured in urine and fecal samples. The samples were decomposed using NaOH, extracted with cyclohexane and diethyl ether, and finally derivatized with diazomethane. PCBT in urine samples was analyzed using a gas chromatograph coupled with a mass selective detector equipped with an electron capture detector (5890-5971A-63Ni, Hewlett-Packard, Palo Alto, CA, USA) (Koss et al., 1976; Koss et al., 1979).
Metabolites were analyzed by administering 14C-labeled PCNB to golden ope fish. Fish (17.5 g) were ground in a mortar with anhydrous sodium sulfate, followed by combustion and radioactive measurement using a liquid scintillation counter. The fish powder underwent three extractions with methanol, then two extractions with ether after slight acidification with HCl. The sample was then alkalized with NaOH and extracted again with ether. Metabolites were separated by thin-layer chromatography (TLC) using Merck Kieselgel F 254 plates. The isolated compounds were identified using gas-liquid chromatography-mass spectrometry (LKB 9000 A, LKB Produkter AB, Bromma, Sweden). The GLC column had a diameter of 4 mm and a length of 2.0 m, packed with Chromosorb W-AW-DMCS 80-100 mesh, 1% Octadecyl Silane (Bahig et al., 1981).
Water and sediment samples from Lake Geneva were collected to measure the levels of HCB, PCTP, and PCNB. Water samples were extracted using polydimethylsiloxane (PDMS) strips, and the compounds adsorbed onto the PDMS were subsequently extracted with pentane. Sediment samples were collected from the top 1-cm layer of sediment, air-dried, and subjected to accelerated solvent extraction according to EPA-823-B01-002 methods. The analysis was performed using Leco Corp’s GC×GC-μECD system (St. Joseph, MI, USA) for initial identification and quantification, and based on the Agilent 7890A GC system (7890A GC, Agilent Technologies, Santa Clara, CA, USA). RTX-1 (30 m, 0.25 mm inner diameter, 0.25 μm film thickness) and RTX-50 (2 m, 0.1 mm inner diameter, 0.1 μm film thickness) columns were used in this analysis (Samanipour, 2015).
Various aromatic benzene thiols in hexane were used to extract whole-cell reaction mixtures from several species of microorganisms, and these methylthio derivatives were then analyzed by gas chromatography using an HP model 5982A GC-MS. Identification of methylthio derivatives was confirmed using retention times and mass fragmentation data (Drotar and Fall, 1985).
Metabolites from the bladder, feces, and bile of F344 rats administered PCNB were analyzed. Following chloroform extraction and methylation using diazomethane, purified substances were separated and analyzed by electron capture gas-liquid chromatography (Varian Vista 6000, Varian Inc., Palo Alto, CA, USA) (Smith and Francis, 1983). Analytical Methods of PCTP above are shown in Table 2.
Sample | Analyte | Sample preparation | Instrumental analysis | References |
---|---|---|---|---|
Human serum feces, urine | HCB metabolites | Alkaline digestion, liquid extraction with benzene | GC-MS | Teng et al. (2017), To-Figueras et al. (1997) |
Rat feces, urine | HCB metabolites | Alkaline digestion, liquid extraction with benzene, derivatization with diazomethane | GC-MS | Koss et al. (1976), Koss et al. (1979) |
Water, fish | PCNB metabolites | Liquid extraction with methanol, acidic, alkaline digestion and ether extraction, TLC chromatogram | GC-MS | Bahig et al. (1981) |
Water, sediment | HCB, PCTP, PCNB | PDMS strip extraction | GC×GC-μECD | Samanipour (2015) |
Microorganisms | Methylthio derivatives | Liquid extraction with benzenethiol, hexane | GC-MS | Drotar and Fall (1985) |
Rat bladder, feces, bile | PCNB metabolites | Liquid extraction with chloroform, derivatization with diazomethane | GC-ECD | Smith and Francis (1983) |
Existing research has focused on detecting metabolites of substances like HCB in environmental samples, human serum, and feces. However, to fully assess the potential risks posed by PCTP, future studies must extend to include food matrices. This expansion is crucial for ensuring comprehensive monitoring across all relevant exposure pathways. Such an approach is essential for accurately evaluating human exposure and protecting public health.
4. Occurrence
PCTP is a compound of concern in the environment due to its toxicity and persistence. Studies have been conducted on its occurrence in various environmental matrices, such as water, sediment, and biological tissues. This section reviews the occurrence of PCTP, focusing on its detection in Lake Geneva and human biological samples.
The occurrence of PCTP in Switzerland’s Lake Geneva has been extensively analyzed. This study collected water and sediment samples in 2011 to measure PCTP concentrations. PCTP was detected in all 30 water samples, with concentrations ranging from 193.7 to 225.2 ng/L. The consistent detection of PCTP in multiple water samples suggests the widespread presence of PCTP in the aquatic environment. Sediment samples from Lake Geneva were also investigated. PCTP was detected in three sediment samples at concentrations ranging from 2.0 to 6.8 ng/kg, while one sample was below the quantification limit (Samanipour, 2015).
The occurrence of PCTP in biological samples has been studied primarily through the measurement of its metabolite, HCB. A study was conducted to measure the concentrations of HCB and related compounds in human biological tissues. This study focused on non-invasive sampling methods by analyzing urine samples from 53 individuals. The average concentration of HCB in the urine samples was 8.8 μg/L, with a range from 0.5 to 86.9 μg/L (Smolders et al., 2009). Although PCTP was not directly measured in this study, the detection of HCB suggests that the study subjects might have been exposed to PCTP. The wide range of HCB concentrations in the urine samples suggests that exposure levels can vary due to environmental factors, lifestyle, and proximity to sources of PCTP. Occurrences of PCTP are shown in Table 3.
Matrix | Sample | n | PCTP | References | |
---|---|---|---|---|---|
Mean | Range | ||||
Lake water | Water | 30 | 193.7-225.2 ng/L | - | Samanipour (2015) |
Sediment | 4 | 3.48 ng/kg | ND-6.8 ng/kg | ||
Human | Feces | 53 | 32.2 ng/g | 5-139.0 ng/g | To-Figueras et al. (2000) |
Urine | 53 | 8.8 μg/24 h | 0.5-86.9 μg/24 h | ||
Urine | 100 | 3.47 μg/24 h | 0.17-84 μg/24 h | To-Figueras et al. (1997) |
The detection of PCTP in environmental and biological samples highlights its widespread presence in nature and raises concerns about its environmental and health impacts. The presence of PCTP in both the water and sediments of Lake Geneva suggests several potential sources of pollution, including industrial discharges or agricultural runoff. The accumulation of PCTP in sediments demonstrates its ability to persist in the environment, posing risks to aquatic organisms and creating a source from which PCTP can enter the food chain.
The presence of HCB in human urine samples indicates that people may be exposed to PCTP through environmental contact or ingestion of contaminated water and food. The variability in HCB concentrations among individuals underscores the need for further research to identify major sources of exposure and to assess the health risks associated with PCTP.
5. Conclusions
This review underscores the environmental and health implications associated with PCTP exposure. Toxicological studies highlight PCTP’s persistence and potential bioaccumulation in aquatic ecosystems and the risks posed to aquatic organisms and food chains. An analysis of analytical methods reveals advancements in detecting PCTP in environmental matrices as well as continued challenges regarding method validation and the need to improve sensitivity.
Occurrence data obtained from Lake Geneva and human urine samples indicate widespread exposure to PCTP, emphasizing the need for continued monitoring and regulatory measures. Understanding the sources and pathways of PCTP contamination is crucial for mitigating its environmental impact and safeguarding public health.
Moving forward, future research should focus on expanding toxicological studies to elucidate PCTP’s mechanisms of toxicity and long-term effects on ecosystems. Improved analytical techniques are still needed and will enhance our ability to quantify PCTP accurately in diverse environmental samples, supporting effective risk assessment and management strategies.
This review contributes to the broader understanding of PCTP by synthesizing current knowledge and identifying key research directions to address knowledge gaps and enhance environmental stewardship and public health protection.