Introduction
Lepidium meyenii Walp. (Brassicaceae), known as maca or Peruvian ginseng, is a perennial herbaceous plant mainly cultivated in the central Andes of Peru at an altitude of 3,500-4,500 m. Maca has been used as a food supplement and traditional medicine for thousands of years. Over the past 20 years, there has been a growing interest in maca and its consumption in different parts of the world, and several commercial maca products have gained popularity as dietary supplements owing to their nutritional and medicinal benefits (Peres et al., 2020).
Air-dried Peruvian maca hypocotyl is rich in nutritional components, such as proteins, carbohydrates, and minerals (Chen et al., 2017a; Dini et al., 1994; Peres et al., 2020). Furthernore, the biological activities of these components, highlight the nutraceutical potential of maca and identified several functional secondary metabolites, have beneficial health effects including macaenes, alkaloids (such as macamides), glucosinolates, sterols, and other components (polysaccharides, polyphenols, and proteins) (Wang and Zhu, 2019). Maca is generally distinguished by color such as yellow, purple, and black, which is linked to significant variations in phenolic compounds and secondary metabolites contents, cultivation conditions, postharvest practice, and analytical methods (Clément et al., 2010; Esparza et al., 2015; Wang and Zhu, 2019; Yábar et al., 2011). Macamides and macaene are used as representative marker compounds for quality control of maca; however, the biologically active components of maca have not been fully elucidated.
Considerable effort has been devoted to extract and identify bioactive compounds and secondary metabolites from maca using conventional and non-conventional methods. From an industrial point of view, the conventional solvent extraction method is preferred owing to factors such as simplicity, efficiency, and cost-effectiveness. To optimize the conventional solvent extraction method, various parameters are recognized as being important, such as sample pre-treatment, combinations of solvents, extraction time and temperature, and solvent-to-solid ratio. However, to date, there is little information on the optimization of solvent extraction and extraction efficiency to maximum nutritional and functional benefits of maca.
In the present study, we examine the solvents suitable for maca extraction using the Soxhlet extraction (SE) and maceration extraction (ME) methods, and investigate the extraction parameters (solvent type, temperature, time, and the solid-to-solvent ratio). Specifically, we focused on the extraction yields of nutritional components (soluble proteins [SPs], total sugars [TSs], and reducing sugars [RSs]), bioactive compounds (total phenolic compounds [TPCs], total flavonoids [TFs], and radical-scavenging activity [RSA]), and macamide B as a marker in the extracts.
Materials and methods
Sun-dried yellow maca roots cultivated in Peru were imported and supplied by SSBIOPHARM Co. Ltd. (Cheonan, South Korea). The root samples were ground to obtain a fine powder using a blender and sieve (1 mm), and then stored at -20°C until analysis.
Analytical-grade organic solvents (95% ethanol, concentrated hydrochloric acid [HCl], glacial acetic acid [AcOH], and n-hexane), and deionized water (water) were used for the extraction. High-performance liquid chromatography (HPLC)- grade solvents (water, methanol, acetonitrile, and trifluoroacetic acid) were purchased from JT Baker Chemical Co. (Phillipsburg, NJ, USA). Standards such as gallic acid for TPC, trolox for RSA, bovine serum albumin (BSA) for SP, and glucose for TS and RS as well as Folin-Ciocalteu reagent to determine SP and TPC and 2,2-diphenyl-1- picrylhydarazyl (DPPH) to measure RSA were procured from Sigma-Aldrich (St. Louis, MO, USA). Quercetin for TF and N-benzylpalmitamide (N-benzylhexadecanamide) (macamide B) were purchased from Cayman Chemical Co. (Ann Arbor, MI, USA). All other chemicals used for analyses were reagent grade and were supplied from Samchun Pure Chemical Co., Ltd. (Gyeonggi-do, South Korea).
The moisture content of the maca root powder was determined by a moisture analyzer (ML-50, A&D Co. Ltd., Tokyo, Japan). The contents of crude protein, crude fat, total ash, and free sugars (maltose, sucrose, lactose, glucose, and fructose) were analyzed according to AOAC methods 981.10, 920.39, 923.03, and 980.13, respectively (Horwitz and Latimer, 2005). The total carbohydrate content was calculated using the following formula: 100 - (weight in grams [protein + fat + water + ash + alcohol] in 100 g of sample) (FAO, 2003).
The extraction of the maca root powder was carried out using water, ethanol (EtOH), acidified EtOH, n-hexane, and mixture of these solvents. For SE, 20 g of maca powder was weighed and dissolved in 200 mL of solvent. In each case, the mixture was refluxed in a glass Soxhlet appartus for 5 h at the boiling point of the solvent. For ME, an appropriate amount of maca powder was suspended in the extraction solvent and continuously stirred using a magnetic stirrer for 5 h at a given temperature. After SE and ME, the volumes of the extracts were measured quantitatively. The mixtures were centrifuged at 13,000 rpm to remove insoluble materials, and the clear supernatants were collected and stored at 4°C for further analyses.
To optimize the simultaneous extraction method, extraction parameters (temperature, time, the solvent-to-solid ratio, and acidified EtOH) were evaluated using a single-factor experimental design. The effects of temperature (25, 40, 50, and 60°C) for 5 h and extraction time (1, 2.5, 5, 7.5, 10 h) at 40°C were evaluated with a solvent-to-solid ratio (mL/g) of 100:10. The effect of the solvent-to-solid ratio (100:1, 100:5, 100:10, 100:20, and 100:30) was investigated at 40°C for 5 h. The effect of HCl (0.1, 0.25, 0.5, 1, and 2 N) and AcOH (1, 2.5, 5, and 10 N) in acidified EtOH was examined at 40°C for 5 h with a solvent-to-solid ratio of 100:10.
The SPs content of the extracts was determined using the Lowry method (Lowry et al., 1951) with modification. An aliquot of the extract and/or diluted extract (0.2 mL) was mixed with 1 mL of Lowry’s reagent. After incubation for 15 min at room temperature, the extract was mixed with 0.1 mL of 50% Folin-Ciocalteu phenol reagent and incubated for 30 min at room temperature. The absorbance of the mixture was measured at 750 nm using a spectrophotometer (Optizen POP, Mecasys Co., Daejeon, South Korea). A calibration curve was prepared using 0-0.5 g/L BSA, and the results were expressed as g-BSA equivalents (BSAE) per 100 g dry weight (DW).
The TS content of the extracts was determined using the phenol-sulfuric method (DuBois et al., 1956) with minor modification. An aliquot of the extract and/or diluted extract (1 mL) was mixed with 1 mL of 5% phenol solution and 5 mL of concentrated sulfuric acid. After incubation for 30 min at room temperature, the absorbance of the mixture was measured at 470 nm. A calibration curve was prepared using 0-0.5 g/L glucose. The RS content of the extracts was measured using the dinitrosalicylic acid method (Miller, 1959) with minor modification. An aliquot of the extract and/or diluted extract (1 mL) was mixed with 3 mL of DNS reagent. After incubation for 5 min in a boiling water bath, the mixture was immediately cooled and mixed with 8 mL water. The absorbance of the mixture was measured at 575 nm. A calibration curve was prepared using 0-1 g/L glucose. The TS and RS measurements were expressed as g-glucose equivalents (GE) per 100 g DW.
The TPC content of the extracts was determined using the Folin-Ciocalteu colorimetric method (Bonoli et al., 2004) with minor modification. An aliquot of the extract and/or diluted extract (0.1 mL) was mixed with 0.5 mL of Folin-Ciocalteu phenol reagent and 6.0 mL of water. After incubation for 2 min at room temperature, the mixture was treated with 2 mL of 15% sodium carbonate and 1.4 mL of water and incubated for 120 min in the dark. The absorbance of the mixture was measured at 755 nm. A calibration curve was prepared using 0-1.7 g/L of gallic acid, and the results were expressed in terms of mg-gallic acid equivalents (GAE) per 100 g DW. The TF content of the extracts was measured using the aluminum nitrate colorimetric method (Alencar et al., 2007) with minor modification. An aliquot of extract and/or diluted extract (0.5 mL) was mixed 1.5 mL of 50% EtOH and then treated with 0.1 mL of 10% aluminum nitrate, 0.1 mL of 1 M potassium acetate, and 2.8 mL of water. The mixture was then incubated for 40 min at room temperature, and absorbance was measured at 415 nm. A standard curve was prepared using 0-300 mg/L of quercetin, and the results were expressed as-mg quercetin equivalents (QE) per 100 g DW.
The RSA of the extracts was measured using the DPPH assay method (Stratil et al., 2006) with minor modification. Briefly, 1 mL of each extract and/or diluted extract was mixed with 2 mL of 0.3 mM methanolic DPPH (1.5 ≤ absorbance ≤ 2.0). After incubation for 30 min in the dark, the absorbance of the mixture was measured at 515 nm. A standard curve was prepared using 0-50 mg/L of Trolox, and the RSA was expressed in terms of mg-Trolox equivalents (TE) per 100 g DW.
Macamide B was analyzed based on a previously described method (Esparza et al., 2015). An HPLC system combined with a photodiode array detector (Atlus™, PerkinElmer, Waltham, MA, USA) was used with a Brownlee choice C18 column (COSMOSIL, 250 mm×4.6 mm, 5 μm, 60 Å pore size, Nacalai Tesque, Inc., Kyoto, Japan). The solvents (A) acetonitrile, (B) methanol, and (C) water with 0.025% trifluoroacetic acid were used at a flow rate of 1.0 mL/min and a column temperature of 40°C. An initial 4-min run was performed with 65:2:33 of A:B:C, followed by a 2-min gradient increase from 65:2:33 to 85:1:14, a 20-min gradient increase from 85:1:14 to 100% A, and a final 9-min with 100% A. Macamide B was measured at 210 nm using an external standard.
All experiments were performed in triplicate, and the results were expressed as mean values±standard deviation. An analysis of variance (ANOVA) was performed, and the mean variation was analyzed using Duncan’s multiple range test (p<0.05). Statistical analyses were conducted using the Statistical Package for the Social Sciences (SPSS) for Windows (version 12.0; SPSS Inc., Chicago, IL, USA).
Results and Discussion
The water content of the maca root powder was 6.69%, which is lower than previousely reported values of 10.40% (Dini et al., 1994) and 8.41% (Chen et al., 2017a). The protein content was 8.25%, which is slightly lower than previously reported values (8.41%-11.6%) (Dini et al., 1994; Chen et al., 2017a). The contents of crude lipid (0.12%) and ash (2.51%) were also lower than those reported in previous studies (0.88%-2.2% and 4.9%-5.0%, respectively) (Chen et al., 2017a; Dini et al., 1994; Peres et al., 2020). The variation in the composition of maca may reflect differences in color, cultivation conditions, postharvest practices, and the analytical methods used (Clément et al., 2010; Esparza et al., 2015; Wang and Zhu, 2019; Yábar et al., 2011). The soluble free sugar content of the powder including fructose, glucose, sucrose, maltose, and lactose, was 22.7%, which is slightly lower than previously reported (29.5%) (Peres et al., 2020). The total carbohydrate content of the powder was estimated to 82.43%, and polysaccharide content excluding free sugars approximately 59.7%, which is close to the hydrolyzable carbohydrate contents (59.0%) reported by Dini et al. (1994).
Most maca root-based extraction methods for food and nutraceutical purposes are performed using aqueous and alcoholic solvents or a combination of the two. In the SE method, n-hexane, EtOH, water, and 1 N HCl in EtOH (1 N HCl-EtOH) were used for boiling, while the ME method was carried out at 40°C using a combination of solvents such as 50% n-hexane with EtOH and 50% EtOH with water. A solvent-to-solid ratio of 100:10 and an extraction time of 5 h were applied. Based on the overall extraction yield, the preferred solvents are EtOH (30.1%) and 1 N HCl-EtOH (29.2%) for SE and 50% EtOH (41.5%), water (38.7%), and 1 N HCl-EtOH (38.5%) for ME (Table 1). In the SE method, using reflux carried out at the boiling temperature of each solvent, the yield of each component (SP, TS, RS, TPC, TF, antioxidants, and macamide B) was significantly higher in the case of the EtOH and 1 N HCl-EtOH extracts compared to the aqueous extracts. Using the ME method carried out at 40°C for 5 h in EtOH, 1 N HCl-EtOH, 50% EtOH, and water, the TPC yield was 3.52, 8.65, 8.96, and 9.19 mg-GAE/g, respectively. The TPC yield in the EtOH/ hydroethanolic extracts was close to the previously reported values in the hydroethanolic extracts (3.56-9.51 mg-GAE/g) (Campos et al., 2013) and the methanol extracts (4.61-5.85 mg-GAE/g) (Clément et al., 2010). The TPC yield in the aqueous extract by SE (2.43 mg-GAE/g) was lower than that obtained by ME (9.19 mg-GAE/g).
In comparison to the SE yield in EtOH, the ME yields of all components were similar in 1 N HCl-EtOH. The yield of macamide B in the ethanolic extract was approximately 84 μg/g (0.24 μmol/g), which is close to that reported for methanol extracts from yellow Peruvian maca (0.07-0.10 μmol/g for Patalá and 0.37-0.66 μmol/g for Alpacayán) (Clément et al., 2010). Macamides are secondary amides composed of benzylamine and fatty acids that vary in terms of hydrocarbon chain length and degree of unsaturation by an amide linkage (Wang et al., 2007). Due to its structure, we did not detect macamide B in the aqueous extracts regardless of the extraction method used. RSA were between 6.88 and 9.58 mg-TE/g in the EtOH and 1 N HCl-EtOH extracts, which is close to previousely reported in the hydroethanolic extracts by Campos et al. (2013) (4.4-14.2 mg-TE/g). However, the RSA in the aqueous extracts was 4.48-5.00 mg-TE/g, which is lower than that reported in a boiled aqueous extract (108.5 mg-TE/g) (Berlowski et al., 2013). The type and composition of the solvent were the most influential factors in the solvent extraction method. Notably, the presence of EtOH in the extraction solvent was crucial for the extraction of bioactive compounds, such as TPC, TF, RSA, and macamide B from the maca root. Thus, the ME method and 1 N HCl-EtOH solvent are selected for optimizing simultaneous extraction from maca.
The effect of temperature on the ME was evaluated in a 5-h extraction process carried out at with solvent-to-solid ratio of 100:10. A maximum temperature of 60°C, which is lower than the boiling point of EtOH, was applied to minimize solvent evaporation during the ME. The extraction yields of each component against the extraction parameters with EtOH and 1 N HCl-EtOH are summarized in Table 2 and 3, respectively. The overall extraction yields in both the EtOH and 1 N HCl-EtOH extracts proportionally increased with an increase in temperature from 25°C to 60°C. Furthermore, the presence of 1 N HCl in EtOH significantly enhanced the yields of SP, TS, TPC, TF, and RSA at elevated temperatures. The TPC yield in the 1 N HCl-EtOH extract gradually increased from 6.78 mg-GAE/g at 25°C to 9.50 mg-GAE/g at 60°C. Thus, higher temperatures were more effective for TPC and TF extraction using 1 N HCl-EtOH. The optimal temperature for TPC extraction is reported to be 70°C; the TPC yield with 59.9% EtOH and a liquid-to-solid ratio of 16.6 was 9.89 mg-GAE/g after 30 min using the response-surface method (Campos et al., 2013). The yield of SP, TS, and RSA in the 1 N HCl-EtOH extract proportionally increased with temperature 25-60°C whereas the RS yield decreased from 0.243 (40°C) to 0.144 g-GE/g (60°C). The decrease in RS may be associated with the degradation and modification of the reducing groups of free sugars in the presence of HCl at a given temperature (Jeong et al., 2011). Macamide B yield in both the EtOH and 1 N HCl-EtOH extracts was approximately 84 μg/g and was independent of the extraction temperature above 40°C. The optimal temperature for ultrasound-assisted extraction has been reported to be 40°C for macamides and macaenes (Chen et al., 2017b). In general, extraction yields are known to increase with an increase in extraction temperature owing to an increase in solubility, diffusion, and mass transfer rates in solid-liquid phase extraction (Richter et al., 1996), alongside enhanced degradation of the plant matrix (Liu et al., 2010) and the disruption of the interaction between phenolic compounds and proteins or polysaccharides (Shi et al., 2003). Considering the overall extraction yield (including RS), the optimal temperature for ME in 1 N HCl-EtOH was 40°C.
In addition to extraction temperature, extraction time and the solvent-to-solid ratio are important parameters those influence yields in conventional extraction. The effect of extraction time on ME was evaluated at 40°C with a solvent- to-solid ratio of 100:10. The overall extraction yield in EtOH and 1 N HCl-EtOH gradually increased depending on the extraction time, and showed a saturation profile after 2.5-5 h. A reduction in the yield of macamide B and RS in the 1 N HCl-EtOH extract was observed with the prolongation of extraction for more than 10 h. This may be related to the degradation of macamide B and the modification of RS in the presence of 1 N HCl at a given temperature. The optimal extraction time for ME was 5 h. In general, extraction yields increase with an increase in the solvent-to-solid ratio owing to the mass-transfer principle. In our experiments, the difference in the overall extraction yields gradually decreased at solvent-to-solid ratios lower than 100:10. The optimal solvent-to-solid ratios for TF and macamide B extraction were 100:10 and 100:10-100:20, respectively, regardless of the solvent used. Based on the negative interaction between the solvent-to-solid ratio and extraction time, the optimal solvent-to-solid ratio for simultaneous extraction was 100:10. This is the same ratio as for the optimal ultrasound-assisted extraction of macamides and macaenes from petroleum ether at 40°C for 30 min (Chen et al., 2017b).
To study the effect of acidified EtOH, different concentrations of HCl and AcOH in EtOH were used in ME. The addition of acid to extraction solvents has been widely used to extract phenolic compounds, flavonoids, and anthocyanins in food processing (Ju and Howard, 2003; Oancea et al., 2012). The overall extraction yield in the acidified EtOH gradually increased with an increase in the normality of the acid (Table 4). EtOH acidified with HCl proportionally enhanced the overall extraction yield from 17.2% (0 N) to 34.6% (0.5 N), and the maximum obtained yield was 42.9% (2 N). We observed a similar trend for the overall extraction yields of SP, TS, RS, and TPC in EtOH acidified with HCl. We observed a positive correlation between RSA and the HCl concentration in acidified EtOH (R2=0.9677). In comparison, the yields of macamide B and TF were independent of the HCl concentration at approximately 84.2±0.62 μg/g and 1.56± 0.09 mg/g, respectively. EtOH acidified with AcOH also proportionally improved the overall extraction yield from 17.2% (0 N) to 25.7% (10 N). The yields of SP and TS increased from 2.15% and 14.7% to 4% and 16.8% with 5 N AcOH and then decreased to 3.8% and 21.0% with 10 N AcOH, respectively. The TPC yields were positively correlated with the AcOH concentration in the acidified EtOH (R2=0.9656). The macamide B yield was independent of the AcOH concentration, approximately 83.1±1.91 μg/g. The RS yield and RSA decreased dramatically in the presence of AcOH in the acidified EtOH. The TF yield in EtOH acidified with AcOH was slightly higher than that in EtOH acidified with HCl. The macamide B yield was independent of the AcOH concentration. We observed that HCl in EtOH as an extraction solvent was more effective in ME extraction than acetic acid, which is consistent with previously published results for blueberry (Oancea et al., 2012) and grape skin (Ju and Howard, 2003). This is because higher concentrations of acid in extraction solvents can cleave the acylated and sugar groups of anthocyanins during extraction. Thus, less than 1% (0.12 N) of 12 N HCl has been recommended for the extraction of bioactive compounds from grape skin (Putnik et al., 2016). Based on our results, however, 1 N HCl in EtOH is deemed optimal in terms of ME yields.
The DPPH RSA of the 1 N HCl-EtOH extract was higher than that of the EtOH extract. As shown in Fig. 1, we observed a significant correlation between the overall extraction yield and RSA in the EtOH and 1 N HCl-EtOH extracts (R2=0.969; p<0.001). Several extracted components, including TPC, SP, TF, and TS can affect RSA. Indeed, the RSA values of the EtOH and 1 N HCl-EtOH extracts were positively correlated with TPC (R2=0.977, p<0.001), TS (R2=0.963, p<0.001), and SP (R2=0.961, p<0.001). These results are in good agreement with previous studies showing that RSA is significantly correlated with the TPCs in maca roots and leaves (Berlowski et al., 2013; Campos et al., 2013; Lee and Chang, 2019) as well as isolated polysaccharides in maca roots (Gicai et al., 2018; Zha et al., 2014). A correlation between TF and RSA has also been reported for methanolic extracts from maca leaves but not roots (Lee and Chang, 2019).
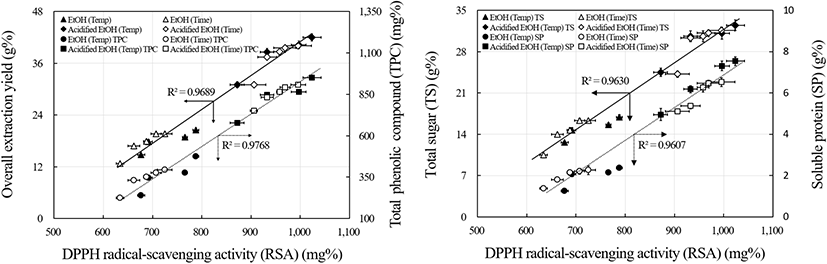
Conclusions
The simultaneous extraction of TPC, TF, SP, TS, RS, RSA, and macamide B from Peruvian maca roots was performed. Optimal extraction using ME was achieved at 40°C for 5 h at with a solvent-to-solid ratio of 100:10 using 1 N HCl in EtOH as the solvent. The addition of HCl to EtOH significantly enhanced the extraction yield of SP, TS, RS, TPC, and RSA based on ME. RSA showed a significant linear correlation with the overall extraction yield; RSA was significantly correlated with TPC, SP, and TS, and was mediated by polyphenolic compounds, polysaccharides, and SP. Further studies are now required to identify SPs and peptides that mediate RSA via synergistic interactions.