Introduction
Peptides derived from various food proteins are considered novel sources of natural antioxidants. These peptides provide nutrition without side effects and help reduce the risk of reactive oxygen species-related human diseases (Zhang et al., 2014). Numerous antioxidant peptides have recently been isolated from food proteins. Such antioxidant peptides are reported from sweet potato protein (Zhang et al., 2014), pecan meal (Hu et al., 2018), rice bran protein (Wattanasiritham et al., 2016), sandfish (Jang et al., 2016), and fish frame protein hydrolysates (Ketnawa and Liceaga, 2017). Most studies on antioxidant peptides have focused on their separation and identification. However, to date, there has been only one study on the relationship between the amino acid composition and biological functions of these peptides (Zhu et al., 2014).
Peptides can be degraded via deamination, oxidation, hydrolysis, and cyclization, which occur during processing and storage. These chemical reactions may lead to the loss of the antioxidant activity of the peptides. Additionally, during gastrointestinal digestion, isolated peptides may be exposed to food-derived atoms and molecules, such as Fe, Cu, hydrogen peroxide, heme, lipid peroxides, nitric oxide, and aldehyde, which may influence the structure of peptides by changing the oxidative conditions (Sannaveerappa et al., 2007; Zhu et al., 2014). The stability of purified peptides must be theoretically and experimentally investigated because the antioxidant activity of isolated peptides can be influenced by several physical and chemical factors. Nevertheless, the influences of various physical and chemical factors on the antioxidant activity of peptides remain unclear.
Perilla (Perilla frutescens var. japonica Hara) is an edible annual plant belonging to the Lamiaceae family that is grown in various Asian countries. Its leaves and seeds are used as fresh vegetable and to make cooking oil, respectively. Perilla is also employed in the production of skin creams, soaps, and medicines because of its recognized broad-spectrum bioactivity, particularly its antioxidant, anti-inflammatory, antiallergic, and antihuman immunodeficiency virus-1 activities (Meng et al., 2009). For instance, perilla oil is reportedly effective in mitigating high blood pressure, thrombosis, and cancer cell proliferation (Asif, 2011). Such benefits have driven the recent increase in the consumption of perilla seed oil as well as the land area used for perilla cultivation (Park and Yoon, 2019a).
Perilla seed meal (PSM), generally considered to be a valueless by-product of the perilla seed oil extraction process, may be a valuable raw material for the production of antioxidant peptides; this is because it is rich in proteins and peptides (Kim et al., 2019). Various studies have investigated the bioactive properties of PSM protein hydrolysates. For example, enzymatic hydrolysates obtained from PSM protein have been shown to exhibit biological activities, such as angiotensin Ⅰ-converting enzyme inhibitory activity, anti-inflammatory, and antioxidant activity (Kim and Yoon, 2020). Park and Yoon (2019a; 2019b) reported that peptide fractions that have been separated from PSM protein hydrolysates via membrane ultrafiltration possess substantial functional properties and demonstrate biological activity.
In our previous study (Kim et al., 2019), two antioxidant peptides (PAP1 and PAP2) were purified from the enzymatic hydrolysate of PSM protein, and their sequences were identified. Although the amino acid sequence of PAP1 could not be confirmed, that of PAP 2 was identified as Ile-Ser- Pro-Arg-Ile-Leu-Ser-Tyr-Asn-Leu-Arg (ISPRILSYNLR, 1330.77 Da). However, the stability of neither of the purified antioxidant peptides was determined. To apply PAP1 and PAP2 as functional food ingredient and nutraceuticals in the food industry, their antioxidant stability under the variable and wide-ranging conditions typically associated with food processing must be confirmed. Thus, in this study, we evaluated the stability of PAP1 and PAP2 under various temperature, pH, and intestinal protease conditions to better assess their potential for application in functional foods.
Material and methods
PSM, supplied by Sunsumi-oil (Namyangju, Korea), was ground and stored in an MDF-435 deep freezer (Sanyo, Tokyo, Japan) at -42°C until use. Trypsin (Novobeta 115) used for hydrolysis was purchased from Novo Nordisk (Bagsvaerd, Denmark). All the reagents used for radical scavenging assays were purchased from Sigma-Aldrich (St. Louis, MO, USA). All other chemicals and reagents were of analytical grade.
PSM protein was obtained by dispersing the PSM in a 1 M NaOH solution (pH 10.0) at 1:10 (w/v). Extraction was performed by stirring the solution for 1 h at 25°C. The pH of the mixture was adjusted to pH 4.0 with 1 M HCl, followed by centrifugation at 1,600 ×g for 30 min. The precipitate obtained via centrifugation was re-dispersed in distilled water, and the pH was adjusted to pH 7.0 using 1 M NaOH. The mixture was then lyophilized and stored at -40°C.
PSM protein was mixed with 0.1 M sodium phosphate buffer (pH 8.0) at 1:20 (w/v) and subsequently hydrolyzed in the presence of trypsin (25 units) over a period of 3 h at 37°C. After the reaction, the mixture was heated in boiling water for 10 min to inactivate the enzyme. The resulting hydrolysate was centrifuged at 1,600 ×g for 30 min, and the supernatant was lyophilized and stored at -40°C until use.
The antioxidant peptides from PSM protein hydrolysate were purified and identified according to the method previously described in our study (Kim et al., 2019). Briefly, tryptic PSM protein hydrolysate was fractionated using an 8050 Amicon stirred ultrafiltration cell (Millipore, Bedford, MA, USA) with three different molecular weight cut-off membranes (3 kDa, 5 kDa, and 10 kDa). The <3 kDa fraction with the highest antioxidant activity was pooled and loaded onto an XBridge OST C18 preparative column (5 μm, 10 mm×250 mm, Waters 2695; Waters Co., Milford, MA, USA) that was connected to an HPLC system. The loaded fraction was divided into seven subfractions using an automatic fraction collector (Teledyne Isco, Lincoln, NE, USA). The fraction that exhibited high antioxidant activity was further purified using an AtlantisTM d C18 column (5 μm, 4.6 mm×150 mm; Waters Co.) connected to the Waters 2695 HPLC system, and single peak was confirmed.
DPPH radical scavenging activity was determined in accordance with a previously described method (Kim et al., 2019). Briefly, 50 μL of 0.2 mM DPPH dissolved in ethanol was added to 100 μL of each sample solution. The absorbance at 517 nm was measured after 30 min of incubation at 37°C. The DPPH radical scavenging activity (%) was calculated as follows: [(1 - (Asample / Acontrol] × 100, where Acontrol is the absorbance of the control (without sample) at 517 nm and Asample is the absorbance of the sample at 517 nm.
ABTS radical scavenging activity was determined in accordance with a previously described method (Kim et al., 2019). Briefly, the ABTS radical cation was generated by mixing 7 mM ABTS stock solution with 2.45 mM potassium persulfate and storing the resultant mixture in the dark for 12 h at 25°C. The ABTS radical solution was diluted in 80% ethanol to an absorbance of 0.60±0.02. Fifty μL of the diluted ABTS radical solution was mixed with 50 μL of the sample. The mixture was left in the dark for 6 min, the absorbance at 734 nm was subsequently measured by using a microplate spectrophotometer (Epoch, Biotek, Winooski, VT, USA). The ABTS radical scavenging activity (%) was calculated as follows: [(1 - (Asample / Acontrol] × 100, where Acontrol is the absorbance of the control (without sample) at 734 nm and Asample is the absorbance of the sample at 734 nm.
The reducing power was determined in accordance with a previously described method (Kim et al., 2019). The sample (100 μL) was mixed with 100 μL of 0.2 M sodium phosphate buffer (pH 6.6) and 100 μL of 1% potassium ferricyanide. The mixture was incubated at 50°C for 20 min. After 100 μL of 10% trichloroacetic acid was added, the mixture was centrifuged at 16,400 ×g for 10 min. One hundred μL of the upper layer was mixed with 100 μL of distilled water and 200 μL of 0.1% ferric chloride; the mixture was kept at 25°C for 10 min. Next, the solution (200 μL) was transferred to a clear 96-well plate, and the absorbance was measured at 700 nm. The results are expressed as absorbance values; a higher absorbance of the reaction mixture indicates higher reducing power capacity.
The purified peptide solution (0.1 mg/mL) was kept in a water bath for 10 min at 30°C, 50°C, 70°C, and 90°C and then cooled to room temperature. The antioxidant activities described above were measured.
After adjusting the pH of peptide solution (10 mL) to one of various pHs (2, 4, 6, 8, 10 or 12) using 1 N HCl or NaOH, the reaction solution was allowed to rest at room temperature for 1 h. The pH of the mixture was neutralized to 7.0 by using 1 N HCl or NaOH; distilled water was added to obtain a 15 mL mixture. The antioxidant activities described above were also measured.
The stability in the presence of various intestinal proteases (pepsin, chymotrypsin, and trypsin) was evaluated in accordance with a previously described method (Jang et al., 2016), with a slight modification in accordance with a report by Lu et al. (2010). Briefly, 0.2 mL of 0.1% sample was mixed with 0.2 mL of 0.01% (w/v) pepsin in the presence of 0.1 M KCl-HCl (pH 2.0) or 0.01% (w/v) chymotrypsin and trypsin in 0.1 M K3PO4 (pH 8.0). The solution was incubated at 37°C for 4 h and then neutralized to pH 7.0 with 0.1 N HCl or NaOH to terminate the reaction. The neutralized solution was centrifuged (10,000 ×g, 30 min), and the antioxidant activity of the supernatant was measured. The stability of the pepsin digest was investigated against continuous digestion by chymotrypsin and trypsin. The pepsin digest was evaporated using the R-124 vacuum evaporator (Buchi, Flawil, Switzerland) and dissolved in 0.2 mL of distilled water. Chymotrypsin and trypsin (0.005% w/v) were added to the mixture and then incubated at 37°C for 4 h. Next, the mixture was adjusted to pH 7.0 with 0.1 N HCl or NaOH to terminate the reaction, and the antioxidant activity was measured.
PAP1 and PAP2 stability under the conditions of heat, pH, and intestinal protease treatments was evaluated by comparing the pre- and post-treatment results. Stability (%) was calculated as (antioxidant activity of sample / antioxidant activity of control) × 100.
All results are expressed as the mean±standard deviation according to the experiments conducted in triplicate. Differences between means of each group were assessed by one-way analysis of variance, followed by the Duncan’s test, both of which were carried out using SPSS ver. 23.0 software (SPSS Inc., Chicago, IL, USA). The statistical significance was set at p<0.05.
Results and discussion
The antioxidant activities of PAP1 and PAP2 were measured and compared with those of the positive controls such as ascorbic acid, catechin, trolox, and butylated hydroxytoluene (BHT). The DPPH radical scavenging activity results are shown in Fig. 1A. The DPPH radical scavenging activities of PAP1, PAP2, ascorbic acid, catechin, trolox, and BHT were 53.8%, 48.13%, 66.36%, 72.38%, 68.34%, and 70.04%, respectively, at 0.1 mg/mL. The DPPH radical scavenging activities of PAP1 and PAP2 were slightly lower than those of antioxidants, but exceeded 48%. As shown in Fig. 1B, PAP1 and PAP2 effectively scavenged ABTS radicals at levels of 67.84% and 78.14%, respectively, at 0.1 mg/mL. As can be seen in Fig. 1C, the reducing power was in the following order: catechin > ascorbic acid > PAP2 > PAP1 > Trolox > BHT. It is worth noting that the reducing powers of PAP1 (0.433) and PAP2 (0.56) at 1 mg/mL were higher than those of trolox (0.4) and BHT (0.262) at 0.1 mg/mL. The results of comparative analyses with the positive controls indicate that the antioxidant capabilities of PAP1 and PAP2 are reasonably high. Thus, purified peptides from PSM protein may be applied as natural antioxidants in the development of functional foods.
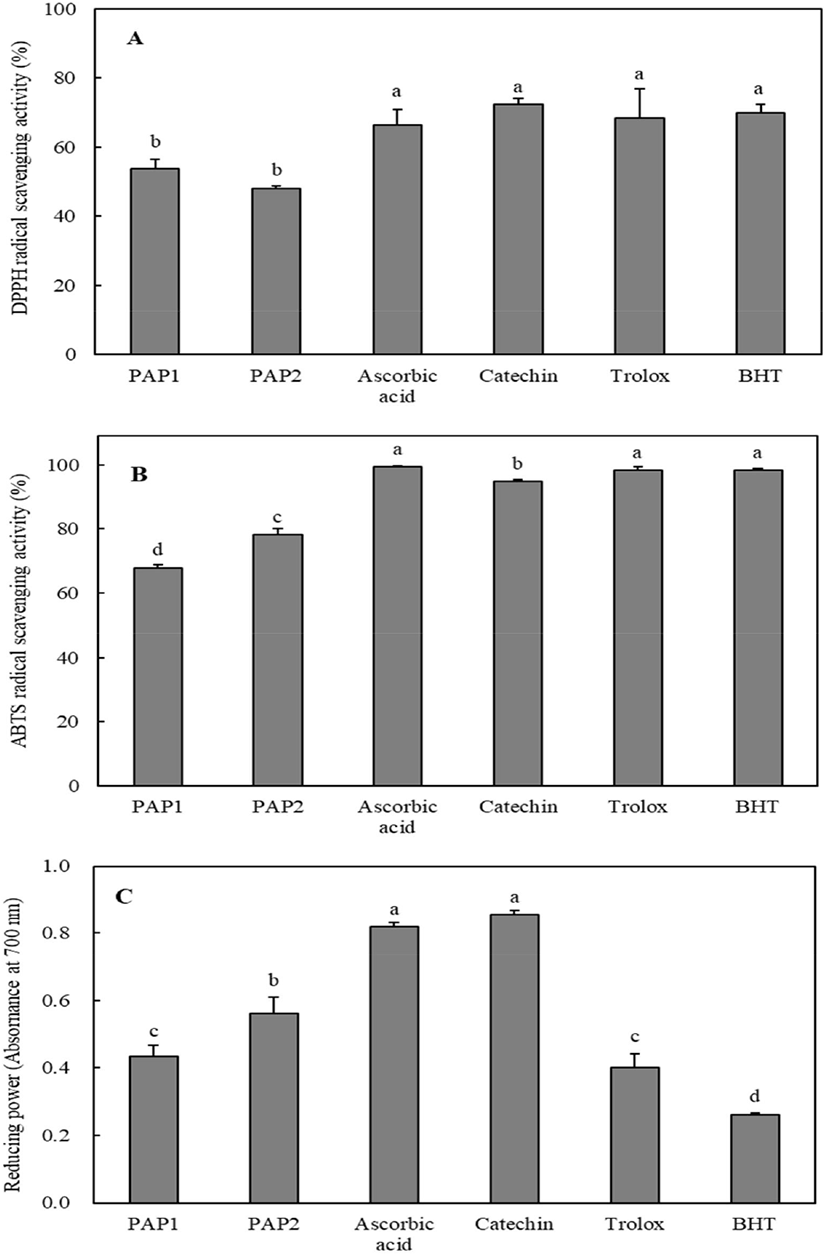
The antioxidant stability of heat-treated purified peptides must be considered in the preparation of food with functional peptides because heat treatment is a common food-processing technology (Djagny et al., 2001; Sheih et al., 2009; Wu and Ding, 2002). To investigate the thermal stability of PAP1 and PAP2, the antioxidant activity measured after they were incubated at 30-90°C was compared to that of the corresponding control, which was not subjected to heat treatment. The antioxidant stability was evaluated by measuring the DPPH and ABTS radical scavenging activity and determining the reducing power. As shown in Fig. 2A, the DPPH radical scavenging activity of the heat-treated PAP1 was not significantly different from that of the control, except in the case of the 90°C treatment. Alternatively, applying a heat treatment to PAP1 slightly enhanced the ABTS radical scavenging activity, indicating better heat resistance. However, the reducing power of heat-treated PAP1 tended to be slightly lower than that of the control; nonetheless, it retained over 90% of its original activity. The antioxidant stability results for PAP2 exposed to different heat-treatment temperatures are shown in Fig. 2B. Although the DPPH radical scavenging activity of PAP2 tended to decrease as the heat-treatment temperature increased, the difference between the values for the control and heat-treated samples was not significant at higher temperatures (i.e., 30°C and 50°C). A similar trend was observed for the ABTS radical scavenging activity. As the temperature increased, the ABTS radical scavenging activity of PAP2 began to gradually decrease; The PAP2 reducing power results revealed that 79.73%, 84.83%, 82.84%, and 84.45% capability was maintained at 30°C, 50°C, 70°C, and 90°C, respectively; these levels were found to be significantly lower than that of the control (p<0.05). These results are consistent with those reported by Wu and Ding (2002), who reported the satisfactory heat resistance of soy-derived biological peptides. In another study, Sheih et al. (2009) found that a peptide from algae protein waste retained all of its bioactivity capabilities, which is consistent with the results of this study. Extremely high temperatures are the primary cause of protein denaturation because they can affect the secondary structure (Gallego et al., 2018; Zhu et al., 2014). Moreover, although most low-molecular-weight peptides do not have tertiary and quaternary structures, they can form secondary structures, which strongly influence antioxidant activity. The purified antioxidant peptides extracted from PSM protein in this study were found to have good thermal stability after being subjected to heat treatments.
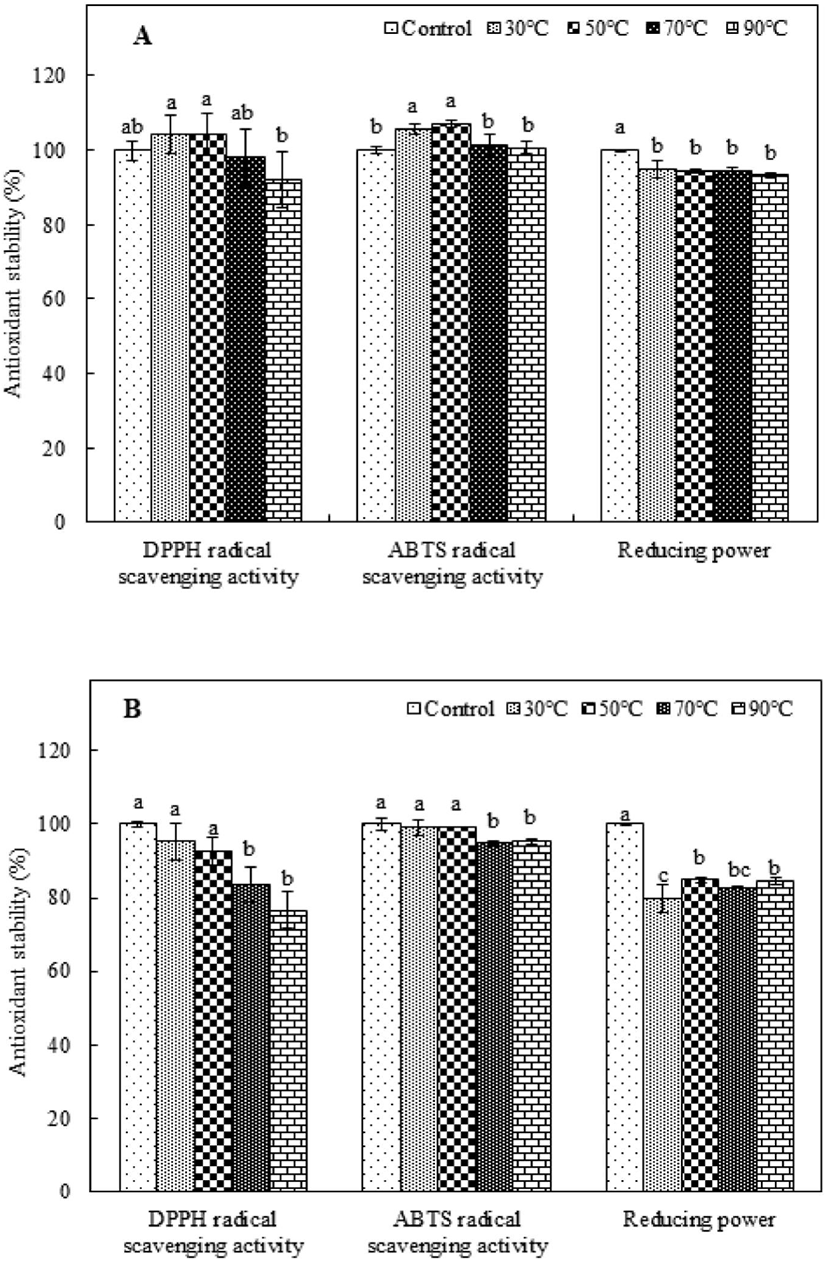
The influence of pH on the antioxidant capabilities of PAP1 and PAP2 was evaluated and compared to the influence on the activity levels of the respective controls, which were incubated without pH adjustment. The results for PAP1 are shown in Fig. 3A. The DPPH radical scavenging activity was maintained at near-neutral pH values (pH 6 and 8); however, the levels of activity were significantly lower (p<0.05) than that of the control under acidic and basic conditions (pH 2, 4, 10, and 12). Conversely, the ABTS radical scavenging activity of PAP1 remained above 90%, i.e., the activity of the control, for all pH values, indicating stability under various pH conditions. Interestingly, the ABTS radical scavenging activity was 101.06% at pH 8. Alternatively, the reducing power results revealed a trend of fluctuation, wherein there was a slight increase at pH 6, 8, and 10, but a slight decrease at pH 2, 4, and 12. The influence of pH on the antioxidant stability of PAP2 can be ascertained from the results presented in Fig. 3B. As the pH value increased from 2 to 6, the retained DPPH radical scavenging activity level increased; however, further increase of the pH was observed to coincide with decreasing retention, indicating less stability under basic conditions (pH 8, 10, and 12). Alternatively, the ABTS radical scavenging activity was not observed to significantly change under the condition of pH 2, 4, 6, or 8, suggesting PAP2 ABTS radical scavenging activity retention under acidic and neutral conditions. Analysis of the reducing power results for PAP2 revealed a trend similar to that of DPPH radical scavenging activity. Specifically, in the case of PAP2, the highest reducing power retention was observed at a pH of 6 (p<0.05); there was less retention under acidic and basic conditions. Nonetheless, the pH stability was evidenced by the consistent values above 70%. The pH is known to influence the electrostatic interactions between charged amino acids. For example, hydroxide and hydrogen ions attract partially charged regions of peptides, disrupting the hydrogen bonds of amino acids. Thus, the structure and conformation of a peptide can change, and its chemical properties can be lost (Jang et al., 2016; Yang et al., 2007). In particular, the structure of a peptide can be easily transformed under alkaline conditions; this could be owing to several factors, such as racemization and deamidation (Zhu et al., 2014). The racemization reaction that can occur in an alkaline environment forms a mixture of L- and D- isomers, which may change the biological activity between isomers. The deamidation reaction is promoted under alkaline conditions; this tends to induce structural and conformational changes and loss of antioxidant activity (Zhu et al., 2014). The low stability of the antioxidant activities of PAP1 and PAP2 that was observed under alkaline conditions is similar to that of an antioxidant peptide that was previously extracted from sandfish protein hydrolysates (Jang et al., 2016). However, the purified peptides that were extracted from PSM protein retained more than 67% of their antioxidant activity under various pH conditions, suggesting that purified peptides from PSM protein hydrolysate may be not be very sensitive to pH.
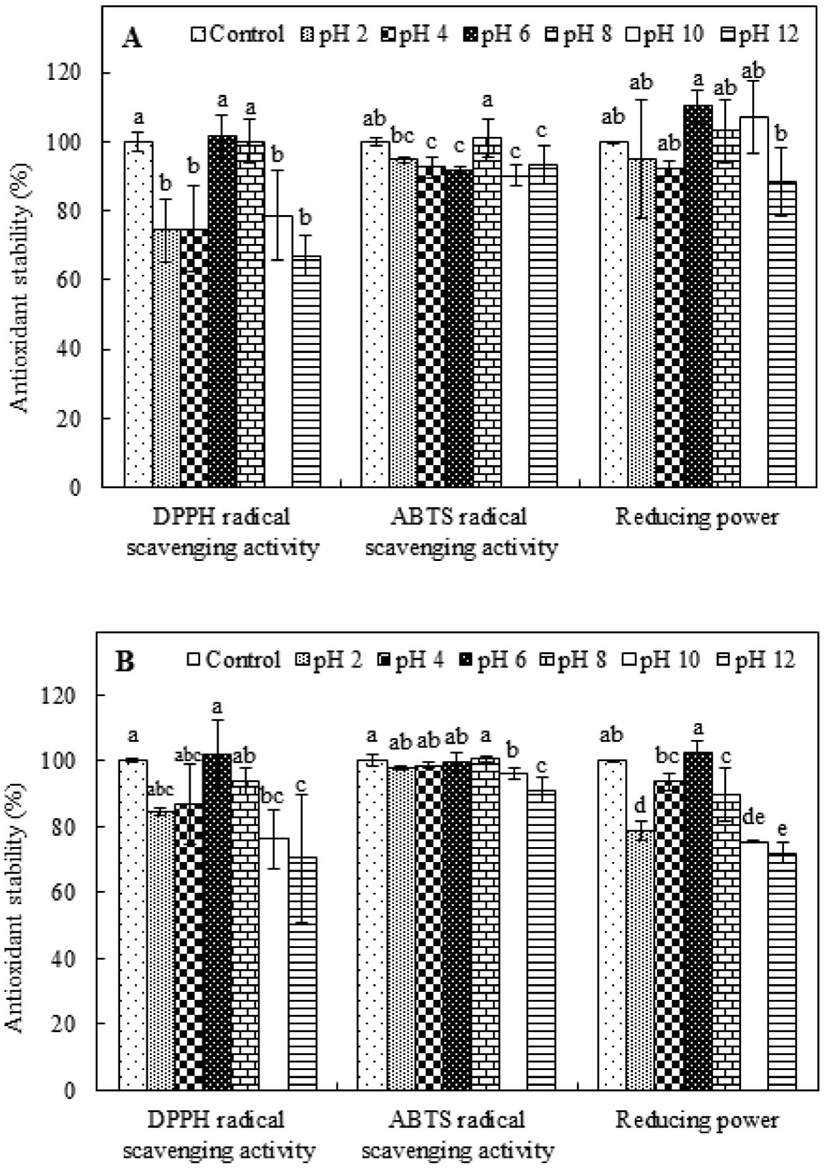
Some food protein-derived biological peptides are believed to fail to exhibit biological activity after oral administration because they undergo hydrolysis in the presence of gastrointestinal proteases (Sheih et al., 2009; Wu and Ding, 2002). Thus, to evaluate the antioxidant stability of PAP1 and PAP2 under the condition of intestinal protease digestion, the purified peptides were treated with various intestinal proteases, i.e., pepsin, chymotrypsin, and trypsin; the antioxidant activity of each PAP type was compared to that of the corresponding control, which was not treated with an intestinal protease. As shown in Fig. 4A, in the case of PAP1, although the level of DPPH radical scavenging activity was reduced by all proteases, it remained above 75%, i.e., the activity of the control. Protease treatment resulted in the retention of or slightly reduced ABTS radical scavenging activity in PAP1. The lowest level of retained ABTS radical scavenging activity was observed for chymotrypsin; the level of activity was 94.19% of that of the control. Alternatively, PAP1 was able to retain most of its reducing power in the presence of pepsin; the value was not significantly different from that of the control. Conversely, the amount of reducing power that was retained by PAP1 in the presence of trypsin was significantly low. In the case of PAP2 (Fig. 4B), the level of DPPH radical scavenging activity was only minimally reduced by each treatment. Furthermore, although lower levels of retained radical scavenging activity were associated with the chymotrypsin and trypsin treatments, there was no signific ant difference relative to the control (p<0.05). The level of retained PAP2 ABTS radical scavenging activity was significantly low for all protease treatments (p<0.05). Nonetheless, the level of scavenging activity was consistently above 66%, i.e., the activity of the control. Additionally, PAP2 was able to retain more than 93% of its reducing power regardless of the type of protease treatment. As an example, 98.63% of the reducing power was retained in the presence of pepsin, corresponding to a value that is not significantly different from that of the control (p<0.05). Many peptides can be broken into smaller-sized fragments under the action of gastric proteases, and eventually completely degraded. Specifically, gastric activity is known to expose internal groups and release free amino acids that influence the size, structure, amino acid composition, and hydrophobicity of peptides, and thus, their antioxidant activity (Gallego et al., 2018; Jang et al., 2016). Our observations are consistent with those reported by Jang et al. (2016), who stated that the level of DPPH radical scavenging activity of a peptide obtained from sandfish protein hydrolysate was reduced by treatment with proteases. Consistency was also observed with the results of a study performed by Gallego et al. (2018), who reported that the antioxidant activity of peptides from Spanish dry-cured ham decreased markedly after in vitro digestion. Nonetheless, in this study, the stability levels of the two antioxidant peptides treated with proteases were found to consistently exceed 66%, indicating that neither antioxidant peptide active structure was fully disrupted after exposure to intestinal proteases.
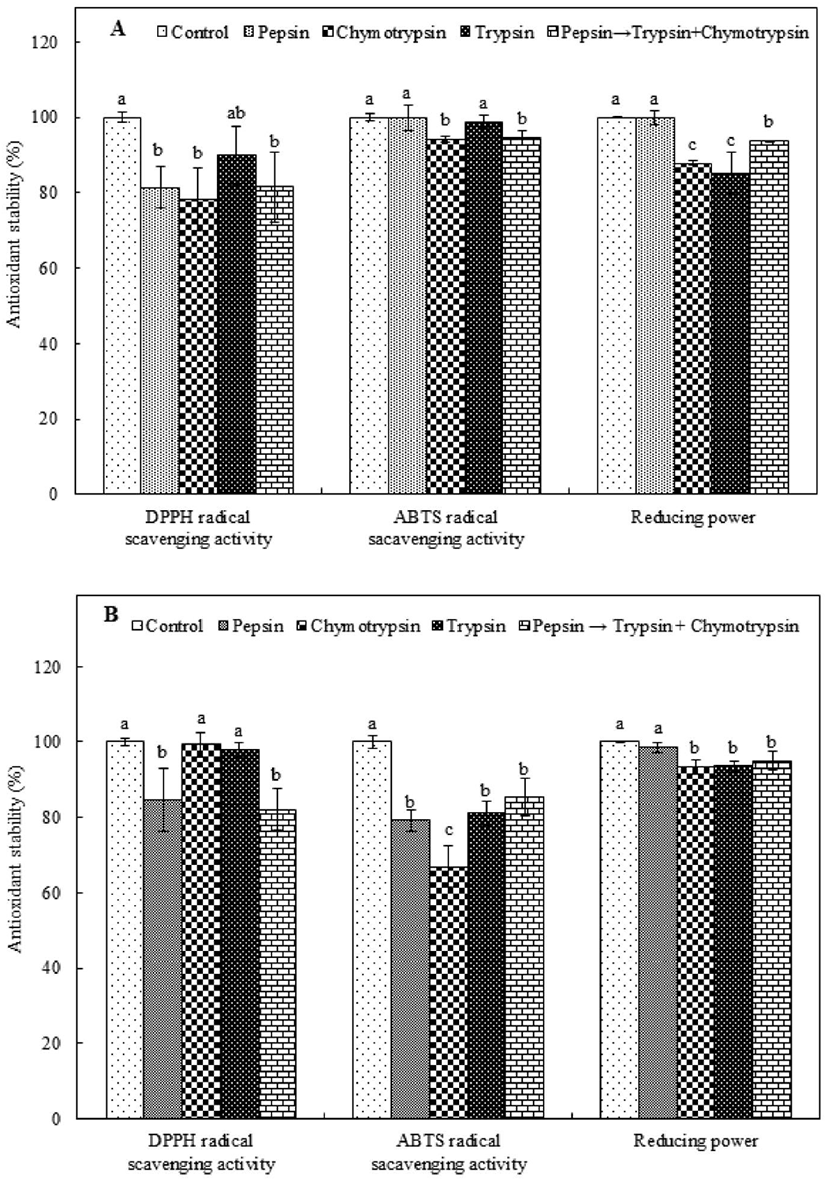
In conclusion, PAP1 and PAP2 exhibited good thermal stability following heat treatment. Alternatively, exposure to strongly acidic or basic environments tended to reduce the antioxidant activity of PAP1 and PAP2. Regarding the protease treatment results, the antioxidant activities of both peptides remained above 66% in the presence of pepsin, chymotrypsin, or trypsin. These results suggest that antioxidant peptides extracted from PSM hydrolysate may be able to maintain their antioxidant activity under variable heat, pH, and intestinal protease conditions, which would allow them to be practically applied in the functional food industry. However, further research is required to determine the bioavailability, potency, and safety of the purified peptides.