Introduction
Eggplant (Solanum melongena L.) is cultivated in different regions of the world, including temperate, tropical, and subtropical zones. As a native plant, eggplant is abundant in India, and different cultivars have varying sizes, shapes, and colors. The composition of eggplant includes various minerals and vitamins of dietary significance such as manganese, copper, and thiamin (vitamin B1) (1). Moreover, eggplant is classified as one of the richest sources of dietary fiber, and is enriched in folate and magnesium. As eggplant is also plentiful in ascorbic acid phenolics, bioactive compounds, and antioxidant compounds, it ranks among the top ten vegetables with the highest radical scavenging activities (RSAs) (2). Eggplant also contains a glycoalkaloid, known as α-solanine, which is considered essential for plant chemical defense. It is well established that glycoalkaloids can be harmful to human health, and such compounds are regarded as causal agents for human intoxication via the inhibition of acetyl cholinesterase (3,4). However, among the phytochemicals in eggplant, α-solanine is classified as an important bioactive compound with several advantageous biological activities such as anti-tumor and antihypertensive activities. Furthermore, eggplant extract also provides atherosclerosis protection and suppresses tumors metastasis (5,6).
Recently, the extraction of phytochemicals from natural plant matrices has progressed owing to the development of more rapid, efficient, and eco-friendly extraction approaches, including microwave-assisted extraction (MAE), ultrasound-assisted extraction, and supercritical fluid extraction (7). Although conventional reflux extraction (CRE) is a well established method, it is time-consuming, uses excess amounts of solvent and energy, and may cause the thermal degradation of thermolabile target compounds from plant materials and their byproducts (leaves, roots, and shoots) (8). In addition, longer extraction time cause increased CO2 emissions to the environment. However, CRE remains the most common extraction method (9). In the last few decades, MAE has emerged as a promising green extraction method owing its advantages, including increased efficiencies for target compound extraction from plant materials, the ability to handle large numbers of samples, shorter extraction times, and reduced energy and solvent consumption with minimal waste generation and CO2 emissions (10). In particular, CO2 emissions adversely affect the climate and are the main cause of global warming, which may cause significant losses to society and the economy. Therefore, the United Nations (UN) General Assembly passed a resolution and stressed that the international community must conform to the UN and Intergovernmental Panel on Climate Change (IPCC) revised guidelines (11).
To obtain the best target responses, it is essential to conduct studies toward the optimization of experimental conditions. Response surface methodology (RSM) is a sophisticated mathematical technique for process and product optimization that analyzes the complex relationship between independent and response variables (12). Among the possible RSM designs, the central composite design (CCD) is the most widely used approach for the statistical optimization of processes. For process and product optimization, RSM provides the additional advantages of low cost and enhanced quality, as it reduces the total number of required experimental runs. During practical RSM optimization, the effects of multiple process parameters are studied while simultaneously varying independent variables (13).
The aim of the present study was to optimize the MAE conditions for eggplant based on the following target responses: Y1: total yield (TY, %); Y2: total anthocyanin content (TAC, mg cyanidin-3-glucoside equivalent (CE)/L); Y3: total phenolic content (TPC, mg gallic acid equivalent (GAE)/100 mL); and Y4, Y5, and Y6: RSAs (1,1’-diphenyl-2-picrylhydrazyl (DPPH), 2,2'-azino-bis-3- ethylbenzothiazoline-6-sulfonic acid (ABTS), and ferric-reducing antioxidant power (FRAP), respectively (mg Trolox equivalent (TE)/100 mL)). The optimum extraction conditions were predicted and verified. Moreover, the extraction efficiencies of the MAE and CRE methods were compared with respect to the target responses, energy consumption, and CO2 emissions.
Materials and methods
Eggplant fruits (Solanum melongena L.) were purchased from a local market in Daegu, cut into cubes (1×1×1 cm), and lyophilized in an MCFD8518 freeze-dryer (Ilsan Boi Base, Insan, Korea). Freeze-dried samples were ground in a lab-scale grinder (FM-909 T, Hanil Electric Co., Seoul, Korea). All regents used were of analytical grade. The ground samples were passed through a 20-mesh sieve to obtain a uniform particle size and then stored at -20oC until further use.
A RSM based on a CCD was used to optimize the MAE process for the target compounds from eggplant. Based on preliminary experiments, three independent process variables were selected for MAE: X1: ethanol concentration (55-95%), X2: microwave power (0-200 W), and X3: extraction time (30-150 s). In total, 16 experimental runs were performed based on the CCD for MAE (Table 1). The target responses recorded in accordance with the CCD are presented in Table 1. Multiple linear regression (MLR) analysis of the experimental results using, SAS software (ver. 8.0, SAS Institute Inc., Cary, NC, USA) gave the following regression equation (Eq. 1):
In addition, to elucidate the interaction effects, predicted model equations were modified to generate four-dimensional (4D) response surfaces using the Mathematica 7.0 program (Wolfram Research, Champaign, IL, USA). Based on the model equations, canonical analysis was used to analyze the maximum and minimum points. In the case of a saddle point (representing a relative minimum or relative maximum), ridge analysis was used to determine the optimum point within the region of interest (14).
MAE was carried out according to the method previously reported by Ameer et al. (7). A Microdigest system (Soxwave 100, Prolabo, Fontenay, France) was used to extract the bioactive components from eggplant powder using the following operational parameters: emission frequency, 2450 MHz and microwave power, 10-250 W. All extraction experiments were performed using the extraction conditions specified by the CCD. The three independent variables were varied in the following ranges: X1: ethanol concentration (55-95%), X2: microwave power (0-200 W), and X3: extraction time (30-150 sec). For each run, an accurately weighed sample (2.5 g) was added to the extraction vessel and mixed with the extraction solvent (50 mL). After extraction, the vessels was cooled to room temperature and the solution was filtered through Whatman No. 41 filter paper. The extraction solvent was added to the final extract to obtain a total volume of 50 mL, and this solution was stored at 4 ± 1℃ until further analysis.
The CRE conditions used in this study corresponded to the optimized reported by Gu (15). CRE was carried out in a water-bath-equipped reflux extractor (C-WBS-D, Changshin Scientific Co., Seoul, Korea) using an ethanol concentration of 75%, and extraction temperature of 80oC, and an extraction time of 3 h. An accurately weighed sample (2.5 g) was mixed with 50 mL of the extraction solvent. After completion of the extraction process, all of the vessels were allowed to cool to room temperature. Then, the extract was filtered through Whatman No. 41 filter paper under vacuum. The filtrate was diluted to 50 mL using the extraction solvent and then transferred to a Falcon tube for storage at 4 ± 1oC until further analysis.
The TY values of the eggplant extracts were determined through a drying method by heating at 105oC, as reported by Kwon et al. (16). The weight difference in the sample was calculated according to the standard method of the Korean food code (17).
The TAC values of the eggplant extracts were analyzed according to the AOAC method (18). First, 0.5 mL of extract was mixed with either two separated solutions were prepared, one with KCl and one with CH3COONa. The absorbance of each solution was measured at 520 and 700 nm (UV-160 PC, Shimadzu, Tokyo, Japan) after 20-50 min. The TAC, expressed as mg CE/L, was calculated using the following equation (Eq 2).
The TPC values of the eggplant extracts were analyzed according to the Folin-Ciocalteu method (19). First, 0.2 mL of each extract was mixed with 1.8 mL of distilled water and then 0.2 mL of Folin-Ciocalteu reagent was added. The extract was allowed to stand for 6 min, and then 2 mL of 7% Na2CO3 was added. The absorbance of this mixture was recorded at 750 nm (UV-160 PC, Shimadzu) after 90 min. The TPC was calculated by comparison with the standard curve of gallic acid (0-20 mg/100 mL) and expressed as mg GAE/100 mL.
The RSAs of the eggplant extracts were determined using various antioxidant assays, namely, DPPH, ABTS, and FRAP assay.
The DPPH RSAs of the eggplant extracts were determined according to the method of Brand-Williams et al. (20). A DPPH solution (Sigma Chemical, St. Louis, MO, USA) was prepared freshly in ethanol and its absorbance at 517 nm was adjusted to 1.000±0.002. Then, 5 mL of the DPPH solution was mixed with the extract (0.5 mL) and the absorbance was measured within 30 s. The ABTS RSAs of the eggplant extracts were evaluated in accordance with the method reported by Re et al. (21). For this purpose, an ABTS solution was freshly prepared used and the absorbance at 734 nm was adjusted to 0.700±0.002. Then, 4 mL of the ABTS solution was mixed with the extraction (0.2 mL) and the absorbance was measured within 5 min. The FRAP activities of the eggplant extracts were evaluated using the absorbance at 593 nm according to the method described by Benzie and Strain (22). Then, 4.5 mL of the FRAP solution was mixed with the extraction (0.5 mL) and the absorbance was measured within 30 min (37℃). A Trolox (6-hydroxy-2,5,7,8-tetramethylchroman-2-carboxylic acid) standard curve was prepared, and the DPPH, ABTS, and FRAP RSAs of the extracts, expressed at mt TE/100 mL, were determined by comparison with the standard curve.
The revised IPCC guidelines (11) were followed to calculate the energy consumption and CO2 emissions. Power and time were multiplied to calculate the power consumption (kWh). Furthermore, the energy consumption, expressed as tons of oil equivalent (TOE), was calculated by employing the fuel calorific value indicated in the Republic of Korea Energy Act; the total calorific value per 1 kWh electricity used is 2,300 kcal. The power consumption was converted into CO2 emissions (TCO2) using the greenhouse gas emissions factor (0.4585 TCO2/MWh) reported by the Korea Power Exchange (MTIE, 2014).
Statistical analyses were performed using Microsoft Office (ver. 2016, Recomond, WA, USA), Mathematica 7.0 program, and SAS software.
The 4D superimposed response surfaces predicted the range of optimum extraction conditions for optimizing TY, TPC, TAC, and the antioxidant activities. Regression equations were formulated by selecting random points within the optimum range. The predicted optimum and experimental values were compared to confirm the reliability of the design (23).
Results and discussion
The effects of three independent variables, namely, ethanol concentration (X1: 55-95%), microwave power (X2: 0-200 W), and extraction time (X3: 30-150 s), on four dependent responses, namely, TY, TAC, TPC, and antioxidant activities (DPPH, ABTS, and FRAP), were investigated. The experimental results from 16 runs, conducted in accordance with the CCD, are summarized in Table 1 in terms of the coded levels of the independent variables. Model equations for the dependent responses (Y1: TY, Y2: TAC, Y3: TPC, and Y4-Y6: antioxidant activities) were developed using regression equations, R2 values, and F-ratio values.
The TY results for the MAE-derived extracts obtained in accordance with the CCD are shown in Table 1 MLR analysis of the experimental data was used to develop, a model for the Y1 response. Significance was ascertained by evaluation of the model coefficients. The determination coefficient (R2) of the model was 0.8877, which implied that the model adequately explained 89% of the variation in TY. A maximum TY of 1.72% was achieved in run number 4, with independent MAE parameters of ethanol concentration (X1): 65%, microwave power (X2): 150 W, and extraction time (X3): 120 s.
The model coefficients were used to formulate the model regression equation for Y1 as shown in Table 2. The F-ratio values for X1, X2, and X3 were 8.29, 0.90, and 3.91, respectively. The lowest TY was 1.19% in run number 12, with independent MAE parameters of X1: 95%, X2: 100 W, and X3: 90 s. The ethanol concentration was the most influential variable among the MAE parameters and significantly influenced the TY (p < 0.05) followed by the extraction time and microwave power (both p < 0.01). Moreover, the maximum morphology for Y1 in the canonical analysis showed a stationary point corresponding to the maximum TY at predicted optimum conditions of X1: 62.40%, X2:173.82 W, and X3: 104.49 s. A 4D response surface plot for TY is shown in Fig. 1(A). The optimum conditions and the predicted levels for the optimum MAE parameters are shown in Table 3. Analysis of the 4D response surface plot was consistent with the canonical analysis. The maximum TY in the 4D superimposed plot occurred at 1.7%, as shown in Fig. 2. Similarly, the TY values of the soluble extracts and saponins obtained from ginseng using MAE with 90% methanol as the extraction solvent, a power output in the range of 75-300 W, and various irradiation times (20, 30, and 40 s) have been determined and the efficiency of MAE compared with that of CRE (16). The authors reported a maximum total soluble extract yield of 30.43% at the optimum MAE conditions of a microwave power of 300 W and an irradiation time of 30 s. Notabley, MAE resulted in a higher extraction yield than time-consuming (12 h) CRE. Moreover, the authors reported that MAE was feasible as an alternative green method for extracting bioactive components from ginseng over a shorter extraction time with reduced energy consumption. Furthermore, the use of a solvent with high polarity led to higher TY values (24).
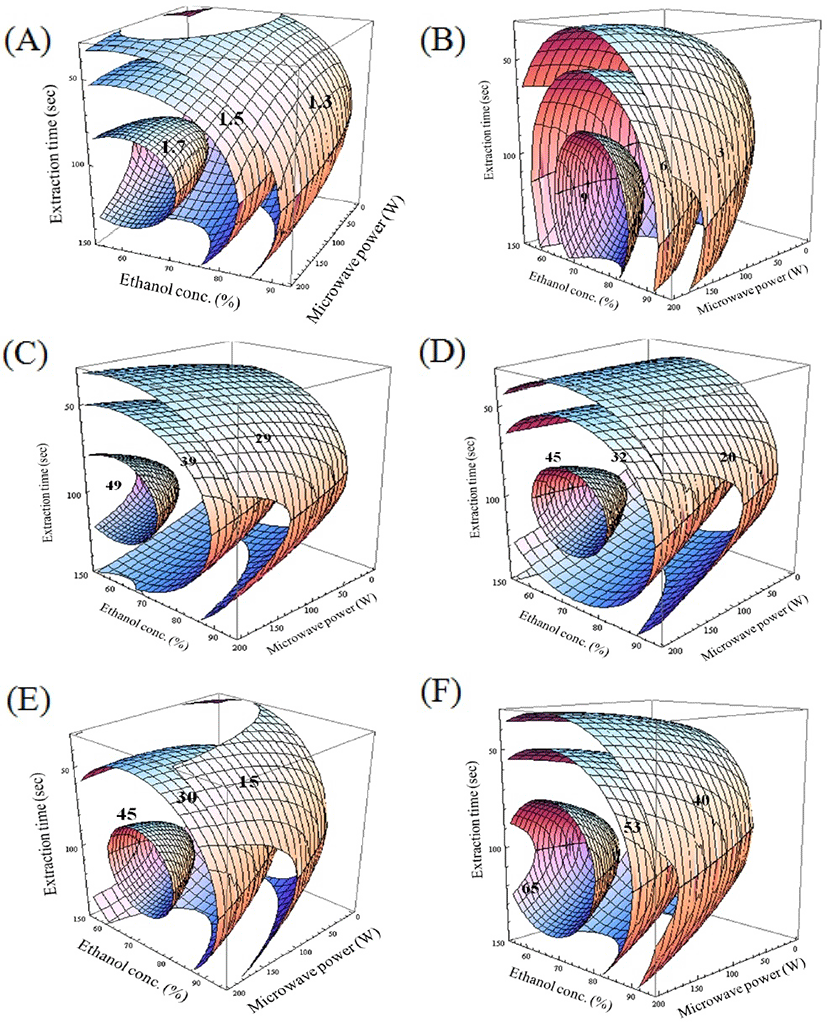
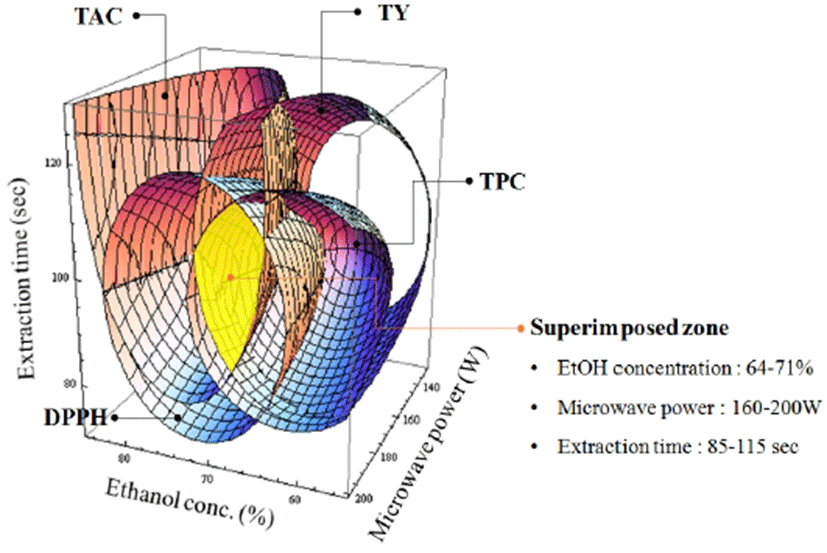
The TAC values of the MAE extracts obtained in accordance with the CCD are summarized in Table 1. MLR analysis of the experimental results was used to develop a model for Y2, as given in Table 2. The model validity was confirmed by the R2 value (0.8723), which indicated that 87.22% of the variability in Y2 could be explained by the developed model with statistical significance (p < 0.0395). The TAC values of the MAE extracts were found to be in the range of 1.23-9.55 mg CE/L. The maximum TAC (9.55 mg CE/L) was obtained in run number 14 under extraction conditions of ethanol concentration (X1): 75%, microwave power (X2): 200 W, and extraction time (X3): 90 s. The stationary point was demonstrated as the maximum point by canonical analysis, and the 4D response surface is shown in Fig. 1(B). The maximum value from the canonical analysis was 10.32 mg CE/L at the predicted optimum MAE conditions of X1: 73.98%, X2: 192.43 W, and X3: 112.68 s. For the Y2 response, the F-ratio values for X1, X2, and X3 were 4.4, 3.39, and 2.95, respectively, and the ethanol concentration was found to be the most influential variable, followed by the microwave power and extraction time. Table 3 shows the optimum conditions and the predicted levels of the optimum MAE parameters. Analysis of the 4D response surface polt was consistent with the canonical analysis. As evident from the 4D response surface plot shown in Fig. 1(B), increases in the ethanol concentration and microwave power led to a corresponding gradual linear increase in the TAC from eggplant. The maximum TAC from the 4D superimposed plot was 9 mg CE/L, as shown in Fig. 2, which is in fair agreement with the experimental value. Similar to our results, the TAC of sweet potato (Ipomoea batatas L. Lam variety, Sinjami) has been optimized by adopting a CCD (25). The maximum TAC from sweet potato was found to be 19.78 mg/100 g under optimal extraction conditions of a drying temperature of 62.91oC, a citric acid concentration of 1.38%, and a soaking time of 2.53 min. The experimental responses showed fair agreement with the predicted response values from the fitted model. Furthermore, Peanparkdee et al. (26) reported that high-polarity ethanol was a suitable solvent for obtaining high-quality extracts enriched in bioactive compounds, TAC, and TPC.
The TPC values of the MAE-derived eggplant extracts obtained in accordance with the CCD are shown in Table 1. The maximum TPC value of 48.75 mg GAE/100 mL was obtained in run number 3 under MAE conditions of ethanol concentration (X1): 65%, microwave power (X2): 150 W , and extraction time (X3): 60 s. MLR was used for model generation and the fitted model equation for the Y3 response is given in Table 2. A high R2 value (96.09%) confirmed the model validity at a significance level of p < 0.05. For Y3 response, the F-ratio values for X1, X2, and X3 were 29.11, 8.39, and 22.79, respectively, and the ethanol concentration was the most influential variable. Table 3 shows the optimum conditions and the predicted levels of the optimized MAE parameters. The maximum morphology was observed and the estimated maximum TPC was 52.42 mg GAE/100 mL at the optimum MAE parameters of X1: 57.46%, X2: 146.83 W, and X3: 96.54 s. The 4D response surface for TPC is shown in Fig. 1(C). The TPC response showed a gradual linear increase corresponding to increases in the independent MAE variables. The maximum TPC from the 4D superimposed plot was 50 mg GAE/100 mL, as shown in Fig. 2, which was in fair agreement with the experimental value. Similar to our results, Maeng et al. (27) optimized the TPC from medicinal turkey tail mushroom (Coriolus versicolor) by RSM. The maximum TPC of 470 mg GAE/100 g DM was obtained at the optimized MAE conditions of an extraction time of 3.8 min, an ethanol concentration of 40%, and a microwave power of 125 W. The authors further reported that TPC showed a gradual linear increase up to an ethanol concentration of 40%, but the TPC decreased at higher ethanol concentrations; furthermore microwave power had the least significant effect among all the independent variables tested. In addition, Teng et al. (28), who determined the maximum TPC content after optimizing the MAE of bioactive components from Chinese quince (Chaenomeles sinensis), found that the ethanol concentration and microwave power were the most influential process parameters.
The RSAs of the eggplant extracts obtained in accordance with the CCD are given in Table 1. The highest DPPH and ABTS RSAs were obtained for the extract from run number 14 (45.95 and 46.74 mg TE/100 mL, respectively) under MAE process parameters of ethanol concentration (X1): 75%, microwave power (X2): 200 W, and extraction time (X3): 90 s. By contrast, the maximum FRAP activity (69.22 mg TE/100 mL) was obtained for the extract from run number 4 at optimized extraction conditions of X1: 65%, X2: 150 W, and X3: 120 s. The minimum FRAP activity was observed in run number 12 under the MAE conditions of X1: 95%, X2: 100 W, and X3: 90 s. Increasing the ethanol concentration up to 65% resulted in a clear increase in the RSAs of the eggplant extracts, but further increases in the ethanol concentration led to a decrease in the RSAs. The F-ratio values for the independent variables (Table 3) demonstrate that the DPPH RSA was most significantly affected (p < 0.05) by both ethanol concentration and extraction time. In the case of the ABTS RSA, all three independent variables equally affected the Y5 response at a significance level of p < 0.01, whereas the FRAP activity was most significantly influenced (p < 0.01) by the ethanol concentration followed by extraction time and microwave power. The model validity was confirmed by the high R2 values for DPPH (90.18%), ABTS (96.98%), and FRAP (94.20%). The estimated maximum DPPH and ABTS RSAs were 48.67 and 48.93 mg TE/100 mL, respectively, at the optimum MAE conditions shown in Table 3. Similarly, the maximum predicted FRAP was 70.61 mg TE/100 mL at the predicted MAE conditions of X1: 68.11%, X2: 191.38 W, and X3: 102.90 s. All three RSAs showed maximum morphologies, and the predicted RSA values were found to be in fair agreement with the experimental values. The response surface plots for Y4, Y5, and Y6 are shown in Fig. 1(D)-(F). The maximum RSA from the 4D superimposed plot was 45 mg TE/100 mL, as shown in Fig. 2, which was in fair agreement with the experimental value. In support of our results, Ilaiyaraja et al. (29) reported the maximum antioxidant activity for the MAE-derived extract of wood apple (Feronia limonia L.). The TPC at the optimized conditions was most significantly (p < 0.05) affected by the ethanol concentration and incubation time. Similarly, Maeng et al. (27) found that ethanol concentration and extraction were the most significant (p < 0.05) independent MAE variables influencing the TPC of extracts from Coriolus versicolor at the optimum MAE conditions. In another study, Singh et al. (30) found that RSM was useful for predicting the optimal phenolic compound contents and antioxidant activity of extracts from potato peel under the optimum MAE conditions of an extraction time of 5 min, an ethanol concentration 100%, and a microwave power 10%. Furthermore, it was found that extracts obtained using solvents with high polarities exhibited the highest RSA values (26).
The optimized MAE method was compared with CRE (15) in terms of the target responses, energy consumption, and CO2 emissions (Table 4). Overall, the extracts recovered by MAE under the optimum conditions showed higher target responses (TY, TAC, TPC, and RSAs) than the CRE-derived extracts. The TY values obtained using MAE (1.66%) and CRE (1.70%) were comparable, whereas the extraction time for MAE was only 100 s while that of CRE was 3 h. Furthermore, the TAC value of the MAE-derived extract was higher than that of the CRE-derived extract, but the TPC values of the extracts were comparable. With regard to the antioxidant activities, the DPPH RSA and FRAP activity were higher in the MAE-derived extract than in the CRE-derived extract, whereas the opposite trend was observed for the ABTS RSA. These results indicate that MAE was more efficient for extracting the desired bioactive components from eggplant then the time-consuming and resource-intensive CRE method. Moreover, MAE consumed much less energy (0.0000011 TOE) than CRE (0.001380 TOE). This higher energy consumption will cause enhanced CO2 emissions. Indeed, as shown in Table 4, the CO2 emissions during MAE were substantially lower (0.000021 TCO2) than those during CRE (0.002751 TCO2). Similar findings have been reported by Ameer et al. (31), who concluded that MAE was a more efficient, eco-friendly approach for extracting steviol glycosides from stevia (Stevia rebaudiana Bertoni) leaf powder than the conventional maceration extraction method.
The demand for eco-friendly extraction methods has risen in the last decade, and MAE shows considerable potential for the green extraction of phytochemicals from plant matrices owing to its short process times and reduced consumption of solvents and, energy. Furthermore, this approach is suitable for automation, offers high reproducibility of food processes with simplified manipulation, provides pollution control accompanied by significant reduction in sample preparation cost and equipment size, and can exploit solvent-free and gravity-induced advanced MAE intensification techniques, such as solvent free microwave hydrodistillation and microwave hydrodiffusion and gravity (32).
Conclusion
Eggplant is a rich source of phytochemicals, including anthocyanin and antioxidant compounds. In this study, the TY, TAC, TPC, and RSAs (DPPH, ABTS, and FRAP) of MAE-derived eggplant extracts were optimized using CCD and RSM based on the following independent variables: ethanol concentration (X1: 55-95%), microwave power (X2: 0-200 W), and extraction time (X3: 30-150 s). The highest TY (1.72%), TAC (9.55 mg CE/L), TPC (48.75 mg GAE/100 mL), and antioxidants activities (DPPH: 45.95, ABTS: 46.74, and FRAP: 69.22 mg TE/100 mL) were obtained at optimal MAE parameters of an ethanol concentration of 70%, a microwave power 160 W, and an extraction time 100 s. Moreover, a comparison of the extraction efficiencies of MAE and CRE revealed that MAE yielded a higher TY, TAC, TPC, and RSAs with a shorter extraction time, lower energy consumption, and lower CO2 emissions. Therefore, RSM allowed the extraction characteristics of individual components to be interpreted depending on the extraction conditions. Furthermore, MAE was shown to be an eco-friendly and efficient method for extracting bioactive compounds from eggplant.