Introduction
Obesity is a disorder wherein excessive body fat accumulates owing to an imbalance in energy expenditure and intake and has become a serious global health concern because it is a risk factor or various severe metabolic comorbidities, including diabetes, cardiovascular injury, hyperlipemia and cancer (1,2). Currently, certain anti-obesity drugs are commercially available, including orlistat and sibutramine. Owing to dissatisfaction with the high costs and potentially hazardous side effects, natural products that can regulate adipogenesis have currently received increasing attention and are an excellent alternative strategy to treat obesity (3,4).
The use of soybean as food products has a long history in Asian countries, and soybeans have a large amount of bioactive compounds and biological activities in addition to their high nutritional value, including estrogenic, antioxidant, anti-osteoporotic, anticarcinogenic, anti-diabetic, and anti-obesity effects (2,5-7). Soymilk is the watery extract of soybeans (8). Fermentation with lactic acid bacteria (LAB) can enhanced not only the physicochemical and sensory properties of soymilk but also the biological anti-obesity effects (9,10). Moreover, isoflavonoids in soymilk are converted from glycosides into the corresponding aglycones after fermentation (11), which display markedly higher absorption efficiency and bioavailability in preventing chronic disease than their glucosides (5).
Fermentation with bacteria of genus Lactobacillus yields conjugated linoleic acid (CLA) from linoleic acid under specific growth conditions (12). CLA has received increasing attention in recent years owing to its potential health effects, including antitumor, anti-atherogenic, antidiabetic, immunomodulatory, osteosynthetic and anti-obesity effects (13). Soybean oil from commodity soybeans contains 54% linoleic acid (14). Soybeans have the potential to produce CLA-rich foods via LAB fermentation.
We previously reported that the antioxidant activity of soymilk was improved upon fermentation with L. plantarum P1201 under optimal conditions and that the fermented soy-powder milk (FSPM) contained high levels of isoflavone aglycones. Moreover, fermentation with L. plantarum can produce CLA, another bio-active substance, in soymilk (6,15-17). In the present study, we aimed to determine isoflavone and CLA content, estimated the inhibitory activity of α-glucosidase, α-amylase, and pancreatic lipase and, the inhibitory effects on adipogenesis in a 3T3-L1 cells for FSPM and unfermented soy-powder milk (UFSPM). We further determined the effects of FSPM and USFPM on the expression of mRNA associated with adipogenesis and lipid metabolism in 3T3-L1 cells to elucidate the underlying inhibitory mechanism.
Methods and materials
The middle soybean cultivar Daewon was obtained from the National Institute of Crop Science (NICS) of the Rural Development Administration (RDA) in Miryang, Korea, in 2013. Safflower seeds were obtained from the Agricultural Processing Office at Hamyang of Gyeongsangnamdo province. Supercritical carbon dioxide fluid (N-TECH, Incheon, Korea) extraction is an important separation technique in food and nutraceutical applications owing to its non-toxic nature (17) and was used to extract safflower seed oil 75 kgf/cm2 and 25±5℃ for 36±12 h. The potential probiotic L. plantarum P1201 was isolated from fermented beverage plant extracts and cultured in an MRS broth/agar (MRSB/MRSA, Difico, Becton Dickinson Co., Sparks, MD, USA) (15). Standard isoflavone aglycone and β-glucosides (daidzein, glycitein, genistein, daidzin, glycitin, and genistin) were purchased from Sigma-Aldrich Co. (St. Louis, MO, USA) and Indofine (Hillsborough, NJ, USA), respectively. Standard CLA, penicillin, and streptomycin were purchased from Sigma-Aldrich Co., Dulbecco’s modified Eagle’s medium (DMEM) and fetal bovine serum (FBS) were purchased from Invitrogen Co. (Grand Island, NY, USA). High-performance liquid chromagraphy (HPLC)-grade H2O, methanol, and acetonitrile were purchased from Fisher Scientific (Fairlawn, NJ, USA). All other reagents are of analytical grade, unless specified otherwise.
Soybeans were washed in tap water for 12 h and drained. Washed soybeans (120 g) were immersed in boiling distilled water for 30 min. The boiled soybeans were dried in a dry oven (HD07026-5003, Hyundae Household Appliances Co., Ltd., Seoul, Korea) at 55℃ for 2-3 days and then pulverized using an ultra-precision grinder into soybean powder. Ten grams of soybean powder was mixed with 100 mL of 2% sucrose in a 250 mL flask. The mixture was sterilized in an autoclave at 121℃ for 15 min. After cooling, the mixture was hydrolyzed with 10 U of cellulase, protease, and esterase at 37℃ for 24 h. Safflower seed oil, at a final concentration of 1.0%, was then added. The pre-culture L. plantarum P1201 was inoculated at a density of 2.0×107 CFU/mL. Fermentation was carried out at 35±1℃ for 48 h (17). UFSPM and FSPM were extracted and stored at -70℃ until analysis.
The pH, acidity and viable cell numbers were determined in accordance with the method of Hwang et al. (15). pH was measured using a pH meter (MP 200, Schwerzenbach, UK). The acidity was determined upon titration with a 0.01 N NaOH solution and was expressed in terms of lactic acid (%). To determine the number of viable cells, 1 mL of the samples was mixed with 9 mL of sterilized distilled water at room temperature (25℃), and the mixture was spread on MRS agar plates. The plate was incubated at 30℃ for 48 h, and the colonies were enumerated. β-Glucosidase activity was evaluated by measuring the hydrolysis ratio of p-nitrophenyl β-D-glucopyranoside (pNPG) in accordance with the method of Hati et al. (8) with some modifications.
The amount of CLA was determined in accordance with the method of Kim et al. (16). Total lipids were extracted using CHCl3. Soybean lipids were mixed with 0.5 N NaOH in methanol. After mixtures were heated at 100℃ for 5 min and cooled to room temperature (25℃), 14% borontrifluoride in methanol (BF3/MeOH) was added and the mixture washed at 100℃ for 30 min for fatty acid methylation. After cooling to room temperature, fatty acid methyl esters were extracted with isooctane and analyzed via gas chromatography (GC) with an SP-2560 capillary column (100 m×0.25 mm i.d., 0.20 μm film thickness; Sigma-Aldrich Co.).
We quantified isoflavones in the UFSPM and FSPM in accordance with the method of Hwang et al. (18). Briefly, the sample (1 g) was extracted with 10 mL of 50% methanol for 12 h at room temperature. The extracts were filtered through a 0.45 μm membrane filter and analyzed via an HPLC system equipped with a LiChrospher 100 RP C18 (4.6×150 mm, 5 μm, Merck KGaA, Darmstadt, Germany). In total 20 μL samples were injected and gradient elution was performed using a two-solvents mixture: 0.1% glacial acetic acid solution (solvent A) and 100% acetonitrile (solvent B). Isoflavones were eluted in accordance with the following program: 0-20 min, 10% solvent B; 30 min, 20% solvent B; 40 min, 25% solvent B; 50 min, 35% solvent B. The flow rate, oven temperature, and detection wavelength were 1 mL/min, 30℃, and 254 nm, respectively.
α-Glucosidase inhibitory activity was determined in accordance with previously described method (17). In total, 50 μL of sample solution, 50 μL of α-glucosidase (0.5 U/mL), and 50 μL of sodium phosphate buffer (200 mM, pH 6.8) were mixed and incubated at 37℃ for 10 min. Thereafter, 100 μL of a 5 mM p-nitrophenyl-α-D-glucopyranoside solution was added to the mixture and was allowed to react for 10 min at 37℃. The the reaction was then terminated via addition of 0.75 mL of 100 mM Na2CO3, and the absorbance of the reaction mixture was measured spectrophotomertically at 420 nm.
α-Amlyase inhibitory activity was assessed in accordance with a previously described method (17). In total, 40 μL of sample solution, 50 μL of α-amylase (0.5 U/mL) and 150 μL of sodium phosphate buffer (200 mM, pH 6.8) were mixed and incubated at 37℃ for 10 min. Thereafter, 250 μL of 1% starch solution in sodium phosphate buffer (pH 6.8) was added to the mixture. The mixture was then allowed to react at 37℃ for 10 min. After having terminated the reaction, 250 μL of a 40 mM 3,5-dinitrosalicylic acid (DNS) solution containing 30% sodium potassium tartrate in 0.5 M NaOH was added, and the mixture was heated for 10 min at 100℃ for color formation. Thereafter, the solution was cooled immediately on ice, and the absorbance was measured spectrophotometrically at 540 nm.
The pancreatic lipase inhibitory assay was performed in accordance with the method of Lee et al. (17). In total, 50 μL of sample solution, 50 μL of lipase (1.0 U/mL) and 50 μL of sodium phosphate buffer (200 mM, pH 6.8) were mixed and incubated at 37℃ for 10 min. Thereafter, 100 μL p-NPB (5 mM) in sodium phosphate buffer (pH 6.8) was added. The mixture was incubated at 37℃ for 10 min. Thereafter, the reaction was terminated via addition of 0.75 mL of 100 mM Na2CO3, and the absorbance was measured spectrophotometrically at 420 nm. A control representing 100% of the enzymatic activity was used similarly; however, conducted the sample solution was replaced with distilled water. Inhibitory activity was calculated as follows:
3T3-L1 cells were purchased from the American Type Culture Collection (Manassas, VA, USA). The subcultured cells were seeded into a 60-mm well plate (5×105 cells/plate) and cultured in DMEM supplemented with 10% FBS and 1% penicillin-streptomycin at 37℃ in a humidified atmosphere at 5% CO2. Upon reaching confluency, the cells were differentiated with a 3T3-L1 differentiation kit (BioVisionInc., Milpitas, CA, USA) containing 1.5 μg/mL in sulin, 1 μM dexamethasone (DEX), 500 μM 3-isobutyl-1-methylxanthine (IBMX), and 1 μM rosiglitazone. After day 3, the differentiation medium was replaced with 10% FBS/DMEM containing 1.5 μg/mL insulin. The medium was replenished every 2 days until 6 day. Samples with different concentrations were administered at the initiation of differentiation and with every medium change for 9 days.
After differentiation ended, cells were washed twice with phosphate-buffered saline (PBS) and fixed with 10% formalin for 60 min at room temperature. The fixed cells were then washed thrice with distilled water (DW) and incubated for 5 min in 60% isopropanol. Isopropanol was eliminated, and the cells were stained with a 0.18% Oil Red O solution in 60% isopropanol for 20 min at room temperature. The Oil Red O solution was eliminated, and the cell culture plate was washed with DW until the excess stain was eliminated. Lipid droplets were stained red. Cell morphology was assessed using an Olympus BX51 microscope (Olympus, Seoul, Korea) with imaging software.
The triglyceride content in adipocytes was determined using a triglyceride colorimetric assay kit (Cayman Chemicals, Ann Arbor, MI, USA). The treated cells were washed thrice with PBS, and the pellet was resuspended in 200 μL of cold standard diluent. The suspension was sonicated for 20 one-second bursts and centrifuged at 12,000 rpm for 15 min. Triglyceride content was determined in the supernatant.
Total RNA was isolated from treated cells, using TRIzol reagent (Invitrogen, Carlsbad, CA, USA), and the RNA concentration and purity were measured using the microplate reader (Infinite M200, Tecan, Salzburg, Austria). cDNA was synthesized using the M-MLV reverse transcriptase kit (Enzynomics, Daejeon, Korea) in accordance with the manufacturer's instructions. The reactions were initiated at 4℃ and held for 5 min. Thereafter, the reactions were subsequently carried out at 37℃ for 60 min and 95℃ for 5 min. Finally, they were held at 4℃ for 10 min.
Lipoprotein lipase (LPL), relative CCAAT enhancer-binding proteins α (C/EBP α), adiponectin, adipocyte fatty acid binding protein (aP2), fatty acid synthase (FAS) and acetyl CoA carboxylase (ACC) mRNA levels were analyzed in adipocytes via RT-PCR, using the Maxime RT-PCR PreMix Kit (Intron Biotechnology, Seoul, Korea) and by qPCR using the TOPrealTM qPCR2×PreMix PCR kit (Enzynomics, Daejeon, Korea) with a 7500 Real-Time PCR System (Applied, Biosystems), with β-actin mRNA used as an internal control (Table 1). Cycling conditions for qPCR analysis were as follows: denaturation at 95℃ for 5 min, followed by 45 cycles of denaturation at 95℃ for 30 sec, annealing at 60℃ for 30 sec (C/EBP-α at 65℃), and extension at 72℃ for 30 sec. mRNA expression levels of all samples were normalized to that of β-actin, and the results are expressed as the fold change of the threshold cycle (Ct) value relative to controls, determined using the 2-△△Ct method. For RT-PCR, the amplified products were analyzed via electrophoresis on a 1.5% agarose gel followed by staining with ethidium bromide.
Data are expressed as the means±standard deviation (SD) values. An analysis of variance (ANOVA), followed by Tukey’s HSD test, was carried out multiple-group comparisons. JMP 10 package (SAS Institute, Carry, NC, USA) was used for statistical analysis, and a p-value <0.05 and a power value of the tests were considered statistically significant.
Results and discussion
In the case of FSPM, a reduction in pH, an increase in the acidity, and an increase in viable cell numbers were observed, compared with those in the case of UFSPM (Table 2). The reduction in pH and the increase in acidity are attributed to the production of lactic acid during fermentation (19). FSPM had appropriate acidity, within the pH range of commercial yogurts (pH 3.9-4.2) (20). Importantly, FSPM exhibited higher levels of β-glucosidase activity than UFSPM, accompanied by a high content of isoflavone aglycones (21).
The total CLA content in FSPM was 1.28 mg/g, among which the cis-9, trans-10 isomer accounted for 90.62%; however, the CLA content in the UFSPM was too low to be detected (Table 3).
Conjugated linoleic acid (CLA) contents (mg/g) | Samples1) | |
---|---|---|
UFSPM | FSPM | |
cis-9, trans-11 CLA | ND2) | 1.16±0.083)a4) |
trans-10, cis-12 CLA | ND | 0.12±0.01b |
Total CLA | ND | 1.28±0.11a |
Microbial fermentation can yield CLA. Van Nieuwenhove et al. (22) reported that the conversion ratio from linoleic acid to CLA by LAB was approximately 17% and 36% in MRS broth and buffalo milk, respectively. Hennessy et al. (23) reported that free linoleic acid was bioconverted to the predominant cis-9, trans-11 CLA isomers by the Bifidobacterium breve and B. longum strains, concurrent with the present results.
As shown in Table 4, the glycoside isoflavone content decreased, while that aglycone isoflavone increased in FSPM compared with UFSPM. β-glycosidase and/or the lactic acid generated during fermentation is involved in the catalysis of β-glycosides into aglycone isoflavone (21,24). These enzymes catalyze the hydrolysis of isoflavone β-glycoside bonds by various LAB. This phenomenon confirms that isoflavone derivatives and their content may be associated with the hydrolases including β-glycosidase effects during soybean fermentation (17). Recent studies have reported an increase in isoflavone aglycones content from isoflavone glucosides by β-glycosidase enzyme in soybeans and soy products. In addition, lactic acid fermentation converts glycoside, malonyl and acetyl glycoside to aglycone (25). Hwang et al. (15) and Lee et al. (17) reported that the daidzein and genistein content increased, while that of daidzin and genistin decreased during fermentation, concurrent with the present results. Aglycone isoflavone has a higher bioavailability than its corresponding glycoside isoflavone (5). Thus, FSPM would be expected to display greater bioactivity than UFPSM.
As shown in Fig. 1, both UFSPM and FSPM inhibited the activities of α-glucosidase, α-amylase, and pancreatic lipase in a dose-dependent manner, owing to isoflavone and phenolic compounds (26-28). FSPM displayed greater inhibition of these enzymes than UFSPM, especially for pancreatic lipase, when treated at the same concentration. α-Glucosidase and α-amylase generate glucose from dietary sources, and the inhibition of both enzymes is considered therapeutic strategy for type-2 diabetes by controlling postprandial hyperglycemia (29). Pancreatic lipase is an important enzyme for digesting dietary triglycerides, and the inhibition of pancreatic lipase can reduce energy intake and prevent obesity (30). Therefore, the inhibition of α-glucosidase, α-amylase and pancreatic lipase by FSPM is considered beneficial to prevent metabolic diseases.
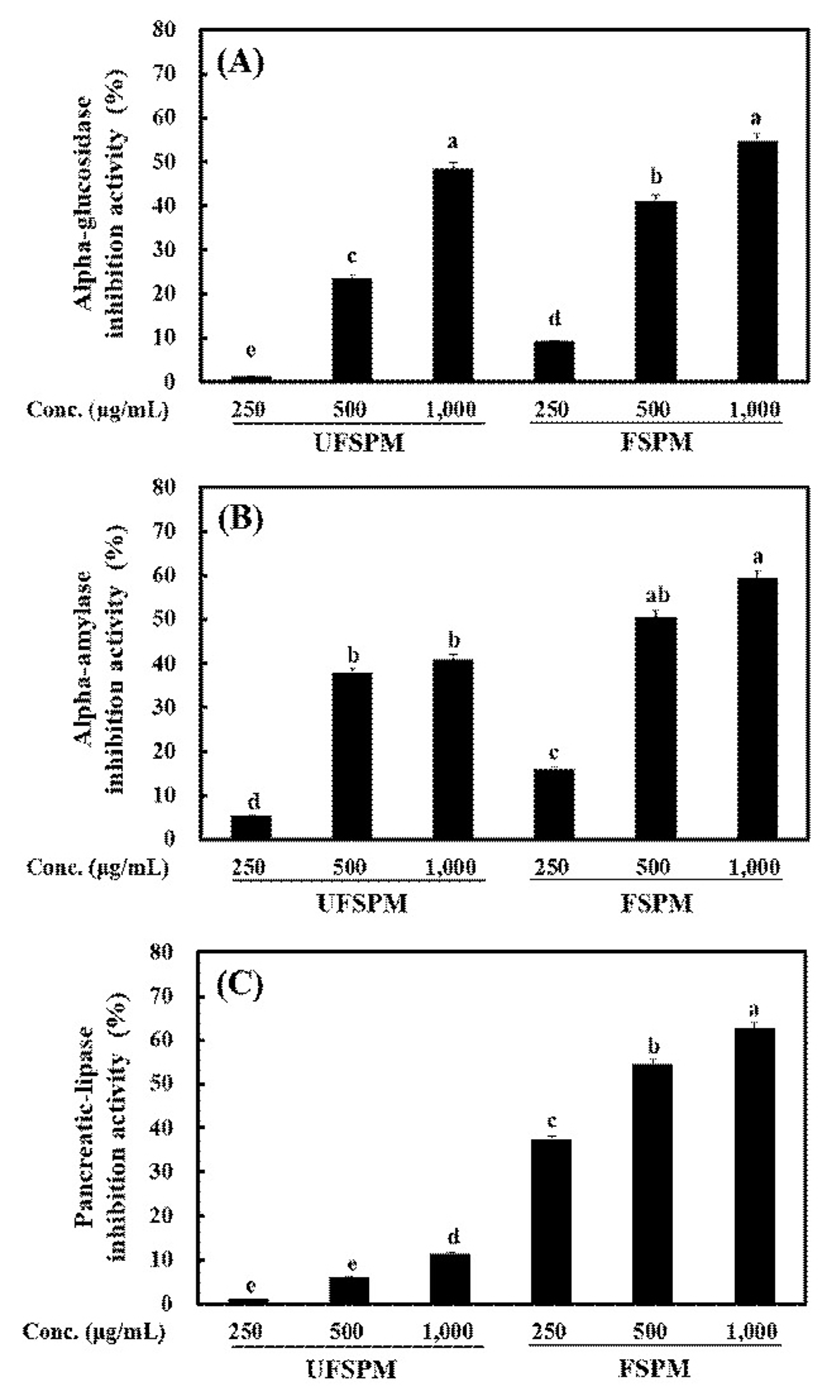
Obesity result from numerous changes in adipocytes through a complex interplay between proliferation and differentiation of pre-adipocytes (31). The inhibition of adipocyte differentiation is a key strategy in preventing obesity. In the presence of a hormonal cocktail comprising IBMX, DEX, rosiglitazone, and insulin, 3T3-L1 preadipocytes reportedly differentiated into adipocyte-like cells and accumulate triacylglycerol-rich lipid droplets (32). To decide the test concentration, the effect of UFSPM and FSPM on the proliferation of 3T3-L1 pre-adipocytes were determined. No toxicity was observed in the concentration range of 100-1,000 μg/mL (data not shown). In the selected concentration range, the effects of UFSPM and FSPM on lipid accumulation in 3T3-L1 cells were investigated. 3T3-L1 cells were differentiated into adipocytes during 9 days in DMEM in the presence or absence of UFSPM and FSPM; thereafter, intracellular lipid content was evaluated via Oil Red O staining. The number of lipid droplets in the FSPM-treated group decreased compared with that of untreated group and UFSPM-treated group (Fig. 2). Moreover, analysis of intracellular triglyceride levels revealed that FSPM caused a dose-dependent reduction in the intracellular accumulation of triglycerides compared to the untreated group. The TG levels decreased by 23.1% at a concentration of 1,000 μg/mL, whereas the UFSPM-treated group did not show a reduction but rather a significant increase in the intracellular accumulation of TG (Fig. 3). These results indicate that FSPM repressed the accumulation of intracellular lipids in adipocytes.
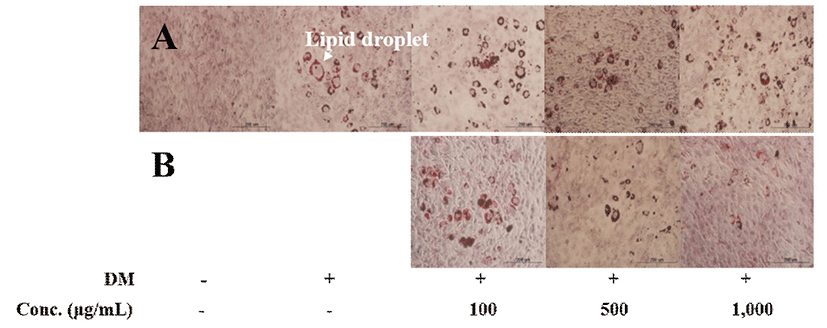
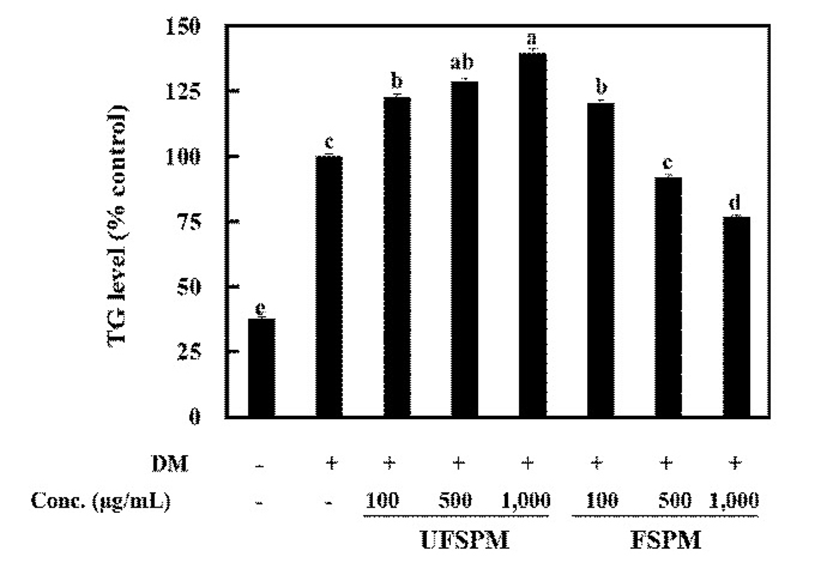
The aglycone isoflavone genistein can efficiently inhibit adipocyte differentiation and has potential anti-obesity effects on 3T3-L1 cells (1). The trans-10, cis-12 isomer of CLA can inhibit preadipocyte differentiation and reduce body fat gain in mice (33). Zhai et al. (34) investigated the effects of trans-10, cis-12 and cis-9, trans-11 isomers of CLA onlipid metabolism in 3T3-L1 cells and reported that the trans-10, cis-12 isomer stimulated adipocyte energy expenditure, apoptosis, and fatty acid oxidation and exhibited a greater effect on lipid metabolism than the cis-9, trans-11 isomer. We attributed the anti-obesity effect of FSPM to aglycone isoflavones and the trans-10, cis-12 isomer of CLA.
To understand the molecular mechanisms underlying the suppression of adipocyte differentiation by FSPM, the expression levels of genes involved in adipogenesis and lipid metabolism were assessed. The mRNA levels of LPL, C/EBP-α, adiponectin, aP2, FAS and ACC in the FSPM-treated (1,000 μg/mL) cells decreased to 43, 39, 81, 25, 32, and 41% of the untreated group, respectively (Fig. 4).
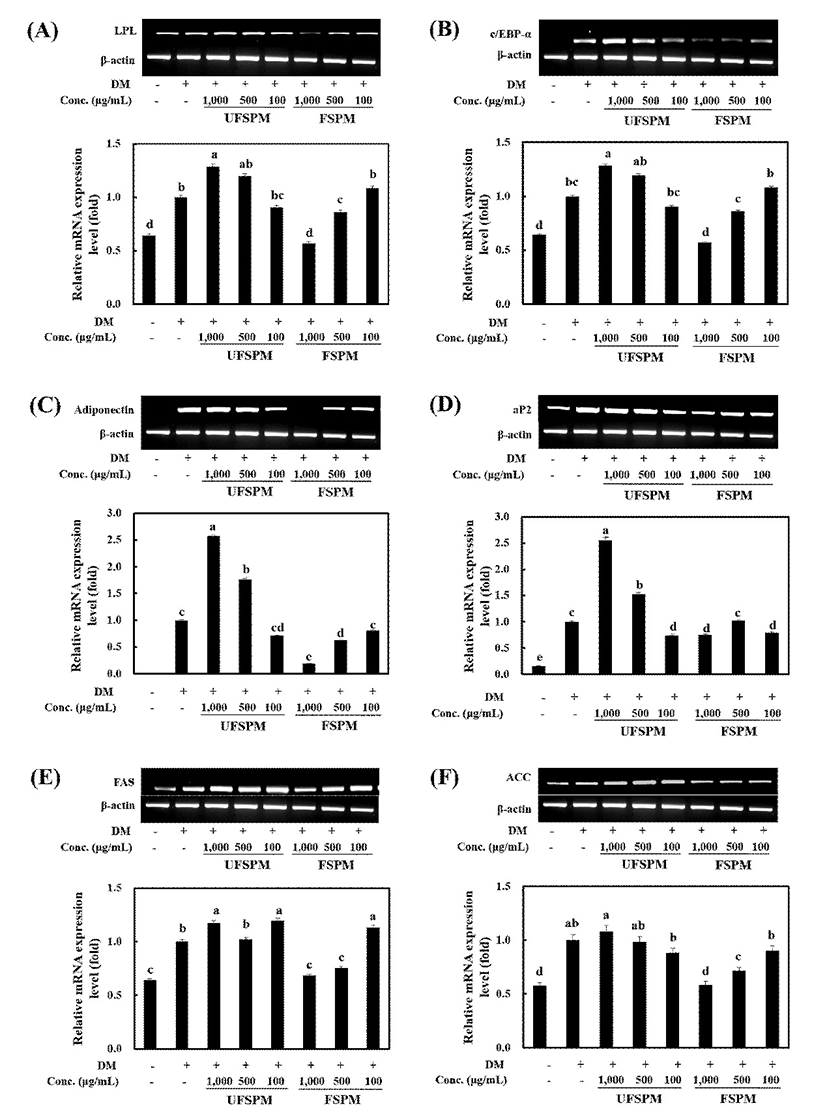
C/EBP-α, one of three isoforms of C/EBPs, is critical activator of adipogenesis and plays an important role in terminal differentiation by maintaining the differentiated status of adipocytes (1). In the present study, C/EBP-α was down-regulated by FSPM. Moreover, target genes regulated by transcription factors, such as LPL, adiponectin, aP2, FAS, and ACC, were also down-regulated. The reduction in LPL mRNA expression reduces intracellular lipid accumulation during differentiation of 3T3-L1 preadipocytes (35). Adipocyte fatty acid-binding protein (aP2) is considered a potential pharmacotherapeutic target because its absence protects against both dietary and genetic obesity (6). FAS is up-regulated in adipose tissue, and inhibition of FAS can inhibit preadipocyte differentiation and suppress food intake, thereby resulting in weight loss in mice (6,36). The lipogenic enzyme ACC yields malonyl-CoA, a substrate of fatty acid synthase, and inhibition of ACC prevents the differentiation of 3T3-L1 cells (37). Adiponectin is the adipocytokine secreted by fully differentiated adipocytes, and its synthesis levels correlate with adipocyte development (35). Down-regulation of adiponectin decreases the adipocyte number. These results indicate that FSPM decreased lipid accumulation in 3T3-L1 adipocytes by suppressing the mRNA expression of genes related associated with adipogenesis and lipid metabolism.
Conclusion
FSPM showed greater inhibitory effects for α-glucosidase, α-amylase, and pancreatic-lipase than UFSPM. Moreover, FSPM inhibited the differentiation of 3T3-L1 cells and decreased lipid accumulation and the triglyceride content in 3T3-L1 cells by suppressing the mRNA expression of genes associated with adipogenesis and lipid metabolism, such as LPL, C/EBP-α, adiponectin, aP2, FAS, and ACC; however, UFSPM did not yield such effects. However, obesity is a complex, chronic metabolic disease. Nonetheless, the results of a 3T3-L1 cell in vitro test were not adequate to prove the anti-obesity effect of FSPM and to understand the anti-obesity mechanism of FSPM. Further studies are required to investigate whether the anti-obesity effect of FSPM persists in an in vivo mouse model of diet-induced obesity.