Introduction
Platycodi radix (PR), the root of Platycodon grandiflorum (Jacq.) A.DC., has been referred to as balloon flower (English), doraji (Korean), kikyo (Japanese), and jiegeng (Chinese). It belongs to the Campanulaceae family and is used as an herbal medicine and food in Asia (1). PR extracts have pharmacological potential for the treatment of inflammatory diseases (2,3). The consumption of PR in Korea was estimated to be greater than 4,000 tons/year and is constantly increasing mainly due to the tendency of consumers to consume healthy diets (4). Generally, PR is vacuum-packed after being cut and peeled before its distribution as a fresh-cut product for the convenience of consumers. These vacuum-packed products have a short shelf life that is mainly attributed to physiological damage as well as biological decays (5). Furthermore, minimally processed PR loses its white appearance as a result of browning on the surface caused after dehydration and lignification after long-term storage at low temperatures, which limits its acceptability to consumers and their desire to purchase it (5). PR is typically consumed raw and mixed with other vegetables or salads, dried or fried vegetables, vegetables soaked in traditional Korean sauces, and mixed vegetables with spices. Because it is usually consumed without cooking, it can contain pathogenic bacteria, a risk to consumer health.
Vacuum packaging and low-temperature storage are general preservation methods that greatly enhance the shelf life and overall quality of fresh products (6). The partial exclusion of oxygen under vacuum packing inhibits the respiratory response of the plant and the growth of spoilage bacteria (7,8). Low-temperature storage is also critical for controlling the survival of mesophilic or pathogenic bacteria (9). Combining low-temperature storage with vacuum packaging could have a synergistic effect on the suppression of microbiological spoilage in food. These conditions inhibit the growth of aerobic microorganisms but can be optimal for the growth of psychrotrophic bacteria (10).
The microorganism levels that determines the shelf life of minimally processed products differs according to the food type and storage conditions. Microbial levels from 5 log CFU/g to 8 log CFU/g are commonly considered convenient quality limits (11). The spoilage of minimally processed products packaged under vacuum conditions occurs when lactic acid bacteria levels exceed 6 log CFU/g (12). Many studies have reported that the dominant bacteria in low-temperature storage are mostly Pseudomonas, Erwinia, Rahnella and Leuconostoc species. These genera contribute to the spoilage of minimally processed products; Leuconostoc is especially important because it is a psychrotolerant lactic acid bacterium and considered to be associated with the spoilage of vacuum-packed products stored at low temperature (13,14).
Total viable counts are a good indicator of the bacterial burden that challenges the preservation of raw materials. However, a more detailed characterization of the microorganisms present in raw materials, or those induced during production steps, distribution and storage, can help trace the causative agents of microbiological spoilage (15). Mass spectrometry-based proteomics using matrix-assisted laser desorption/ionization-time of flight (MALDI-TOF MS) has emerged as a reliable tool for bacterial identification (16-18). This technique can accurately characterize bacteria, with potential identification to the genus, species, and subspecies levels for some taxa (19,20).
The aim of the present study was to investigate microbiological quality and the bacterial communities present in minimally processed PR, as well as the changes in dominant bacterial communities during storage at low temperatures using culture methods and MALDI-TOF mass spectrometry. The knowledge of dominant bacterial communities will provide important information to delay the deterioration of minimally processed PR during long-term storage.
Materials and methods
Minimally processed PR samples were obtained from two manufacturing companies (companies I and II) located in Gyeonggi province. Peeled whole PR was used as a raw material and immersed in tap water for 5 h to eliminate its bitter taste prior to shredding. The washing steps of company I and company II differed in a few ways. PR from company I had been washed three times in running tap water after shredding, whereas that from company II had been dipped in running tap water for 5-10 min before packaging. Samples were collected after shredding, washing, and packaging from the same lot to assess changes in microbiological quality tthat are associated with processing. These samples were placed in sterile bags (Nasco Industries, Fort Atkinson, Wisconsin) and transported at 5℃ to the laboratory.
The end-products for the assessment of microbiological quality during storage were weighed to approximately 250 g, after being washed and sealed using a vacuum packaging machine (SB 260, TURBOVAC, Howden Food Equipment, Holland) from company I. The end-products were transported at 5℃ to the laboratory and stored at 5 and 15℃. Microbiological quality was analyzed in duplicate at approximately 4 day intervals for 5℃ storage and 2 day intervals for 15℃ storage from the beginning of storage to compare samples before and after storage.
Microbiological procedures recommended by the Korean food code (21) and ISO-4833 (22) were performed. Samples were collected from three different processing steps and analyzed for total viable cells (TVCs), coliforms, fungi, Enterobacteriaceae, and two pathogens (Bacillus cereus and Clostridium perfringens). These analyses were performed in duplicate. For this, 25 g of each sample was diluted in 225 mL of 0.85% saline and homogenized using a Stomacher®-400 (Seward, Norfolk, UK) for 2 min at 230 rpm. Ten-fold serial dilutions were made in 0.85% saline, and 1 mL of each dilution suspension was plated for the enumeration of microorganisms using PetrifilmTM plates (3M Microbiology, St. Paul, MN, USA) for TVCs, fungi, and Enterobacteriaceae or Sanita-kun plates (Sanita-Kun, Tokyo, Japan) for coliforms. These plates were incubated at the optimal temperature for each microorganism, and typical colonies were enumerated. B. cereus was isolated by plating the dilution suspension on mannitol-egg yolk-polymyxin B agar (Merck, Darmstadt, Germany) and the plates were incubated at 30℃ for 18 h. C. perfringens was isolated by plating the dilution suspensions on a thin layer (15 mL) of tryptose-sulfite-cycloserine (TSC, Oxoid, Basingstoke, UK) agar without egg yolk. The TSC agar plates were then overlaid with an additional 5 mL of TSC agar. The plates were incubated for 24 h at 37℃ in an anaerobic jar using Genbox packages (BioMérieux, Marcy-l'Étoile, France). The presumptive positive isolates of B. cereus and C. perfringens were subcultured on tryptic soy agar (TSA, Merck) and confirmed biochemically using a VITEK®2 Compact instrument (BioMérieux). The results are expressed as colony-forming units per gram (CFU/g).
Samples, including raw material from company I, were collected after being shredded, washed, and packaged to evaluate changes in dominant bacterial communities during processing. End-products were analyzed at days 0, 3, 7, 12, and 20 when stored at 5℃ and days 0, 1, 3, and 7 when stored at 15℃ to assess changes over the course of storage. Specifically, 25 g of each sample was stomached using a Stomacher®-400 (Seward) with 225 mL of 0.85% saline for 2 min at 230 rpm. Ten-fold dilutions were prepared with 9 mL of 0.85% saline, and 100 μL of each dilution suspension was spread onto plate count agar (PCA, Merck). The plates were then incubated at 37℃ for 24 h, and each colony was then picked for identification. The collection of single colonies for MALDI-TOF MS was carried out using the method described by Holl et al. (23) All colonies from replicate countable plates of each sample were selected. Thus, 200 to 350 colonies was identified from only one sample. For plates with >100 colonies, all colonies growing in a sector comprising half or a quarter of the plate were picked for identification and at least two parts were selected to comprise the representative groups of the major population in bacterial communities. All tests were performed in duplicate to obtain reliable results. The picked colonies were transferred to TSA medium (Merck) and incubated at 37℃ for 24 h. Fresh colonies were directly smeared onto the sample spots of disposable polymeric FlexiMass-DS target slides (Shimadzu-Biotech, Tokyo, Japan). Then, 1 mL of VITEK MS-CHCA matrix solution (10 mg/mL α-cyano-4-hydroxy-cinnamic acid (CHCA), BioMérieux) was applied to the top of the sample and air dried until the matrix and sample co-crystallized. The target slide with all prepared samples was then loaded into the VITEK MS system so that the mass spectra of whole-bacterial cell proteins could be acquired for each strain; these proteins mainly consist of ribosomal proteins. Finally, the mass spectra acquired for each sample were compared to known mass spectra in the database within IVD software and given a confidence score according to how close the acquired spectra matched those contained in the database. Escherichia coli ATCC 8739 was used as the quality control strain. The identities of the dominant bacteria were confirmed biochemically using a VITEK®2 Compact (BioMérieux).
Results expressed as CFU/g were converted to decimal logs and treated by one-way ANOVA using SPSS software (IBM©ÏSPSS Statistics ver. 20, New York, NY, USA) to determine whether microorganism levels differed significantly during processing and storage. A significance level of 0.05 was used for all statistical analyses.
Results
PR was collected at each step from two companies to determine the step that can induce increased bacterial growth during processing. TVCs, fungi, Enterobacteriaceae, coliform, B. cereus, and C. perfringens levels were measured for microbiological quantification (Table 1). For product from company I, the TVCs in raw PR was 5.2 log CFU/g and increased to 5.6 log CFU/g after shredding. At the washing and packaging stages, this level was 1 log CFU/g greater than that of raw PR (i.e. TVCs were 6.2 log CFU/g at the washing step and 6.1 log CFU/g at the packaging step). The fungi level increased from the 3.0 log CFU/g in raw PR to 4.6 log CFU/g after shredding and was maintained at this level until packaging for end-products. Enterobacteriaceae and coliform levels at shredding were 4.5 log CFU/g and 3.5 log CFU/g, respectively, and these levels did not significantly differ from the values in raw PR (4.5 log CFU/g and 3.6 log CFU/g, respectively). The Enterobacteriaceae level increased by 1.0 log CFU/g after washing, reaching 5.5 log CFU/g, whereas the coliform level increased by 0.9 log CFU/g to reach 4.4 log CFU/g. B. cereus and C. perfringens levels were less than 1.0 log CFU/g in all samples collected during processing. For product from company II, the TVC in raw PR was 6.8 log CFU/g; that level was maintained during processing and reached 7.0 log CFU/g in the end-product. The fungi level increased to 4.2 log CFU/g after shredding from 3.8 log CFU/g in raw PR, and that level was maintained to the end-product. Thus, processing did not significantly change the fungi level. Enterobacteriaceae and coliform levels were also not changed by processing; approximately 6.0 log CFU/g was measured in raw PR for both, which was maintained to the end-product. B. cereus levels were 1.5 log CFU/g and 1.2 log CFU/g in raw and shredded PR, respectively, but those levels dropped to less than 1 log CFU/g after dipping. The level of B. cereus in the end-product was also less than 1 log CFU/g. C. perfringens levels were less than 1.0 log CFU/g in all samples collected during processing.
The microbiological quality of end-products stored at 5℃ for 20 days are presented in Fig. 1A. During storage at 5℃, TVCs increased only slightly from the initial day (6.1 log CFU/g) to reach its maximum level (approximately 7.0 log CFU/g) after 5 days and then remained constant throughout the duration of storage. Fungi levels in samples stored at 5℃ did not increase until day 5 but were the highest (5.3 log CFU/g) between 5 and 7 days. Fungal growth decreased after 7 days and reached its minimum of 2 log CFU/g at 20 days. Levels of Enterobacteriaceae and coliforms trended in a similar manner. The level of Enterobacteriaceae increased by 1 log CFU/g from day 0 to day 5, peaking at 6.6 log CFU/g for the entire storage period. However, this level began to decrease at day 5 and reached its minimum (1.4 log CFU/g) 5.4 log CFU/g at day 3 from 4.4 log CFU/g at day 0 and remained at this level between days 3 and 9. The growth at the end of storage. The level of coliforms increased to of coliforms decreased after day 9 of storage and almost reached its minimum of 1.7 log CFU/g at day 20. B. cereus and C. perfringens levels during storage at 5℃ were undetectable (<1 log CFU/g).
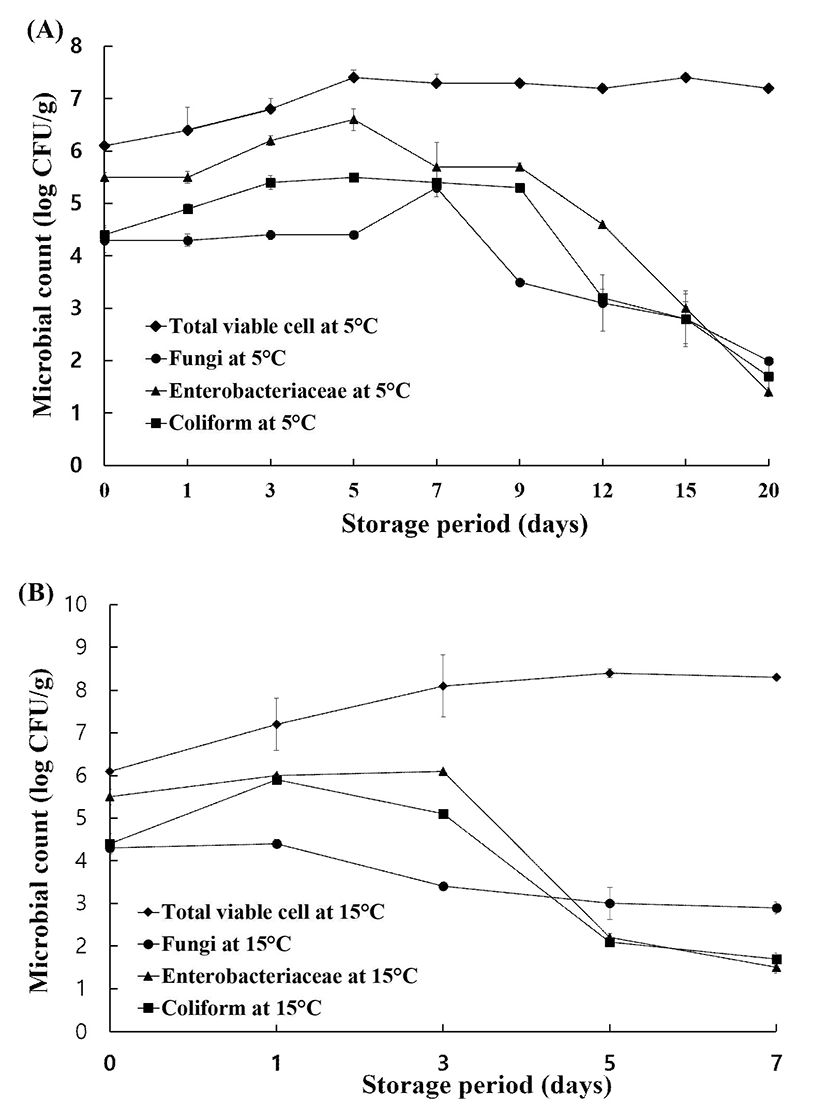
The microbiological quality of the minimally processed PR is presented in Fig. 1B. TVCs increased from 6.1 log CFU/g to its maximum of 8.1 log CFU/g after only 3 days, and this level was maintained until the end of storage (day 7, 8.3 log CFU/g). The fungi level gradually decreased from 4.3 log CFU/g at day 0 to 3.4 log CFU/g at day 3 and 2.9 log CFU/g at day 7. The levels of Enterobacteriaceae and coliforms increased between the initial day and day 3, reaching 6.1 and 5.1 log CFU/g, respectively, after 3 days. For Enterobacteriaceae and coliforms, levels of approximately 2.0 log CFU/g were detected after 5 days, but the minimum values, specifically, 1.5 log CFU/g for Enterobacteriaceae and 1.7 log CFU/g for coliforms, were reached at the end of storage.
A total of 783 microbial colonies were isolated from four different sampling points and identified using culture and MALDI-TOF MS (Fig. 2). The major bacteria isolated from the overall process were Pseudomonas fluorescens, Rahnella aquatilis, Serratia fonticola, Serratia liquefaciens, and Pantoea agglomerans. However, the composition of the bacterial communities exhibited significant differences based on processing step. P. fluorescens was present at the highest relative abundance (47.7%) in raw material, followed by S. liquefaciens (27.9%), R. aquatilis (9%), P. agglomerans (7.2%) and to a lesser extent (<5%) S. fonticola, Carnobacterium maltaromaticum, and Hafnia alvei. At shredding, R. aquatilis was present at the high relative abundance of 39.2%, which was followed by S. liquefaciens (23.0%), P. fluorescens (17.6%), and L. citreum (10.8%). The abundant bacteria groups at washing were P. fluorescens, R. aquatilis, S. fonticola, and S. liquefaciens; and S. liquefaciens was at it's the highest relative abundance among dominant bacteria at the washing step. S. fonticola was the most dominant bacteria (43%) in the end-product, with R. aquatilis, L. pseudomesenteroides, and P. fluorescens present at relative abundances of 23.8, 9.8, and 9.1%.
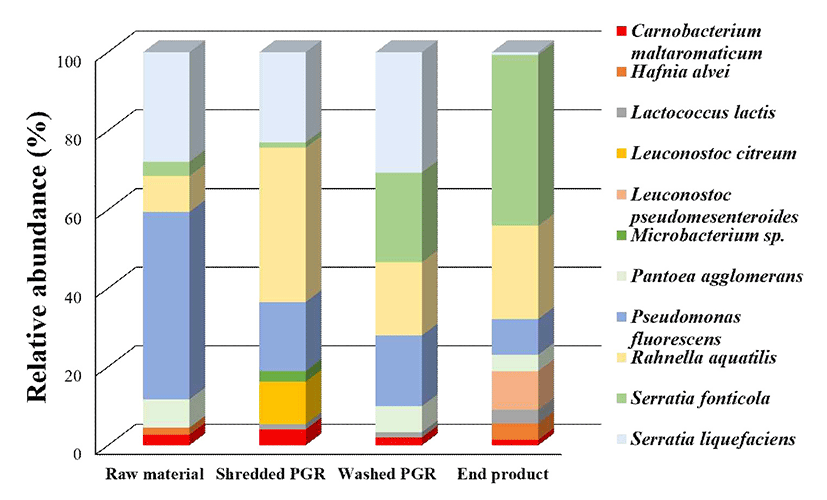
This study also examined the changes in dominant bacterial communities in vacuum-packaged PR during storage. Fig. 3A shows that dominant bacteria isolated from samples stored at 5℃ were quite different before and after storage at 5℃. The relative abundance of L. pseudomesenteroides increased from 9.8 to 55% after 3 days and was present at the highest relative abundance at the end of storage (100%). In contrast, the relative abundance of R. aquatilis decreased from 23.8 to 6% between day 0 and day 7 while stored at 5℃, and this species was not detected from day 12 onward. The relative abundance of S. fonticola trended similarly to that of R. aquatilis, decreasing from 43.4% on day 0 to 0% after day 20.
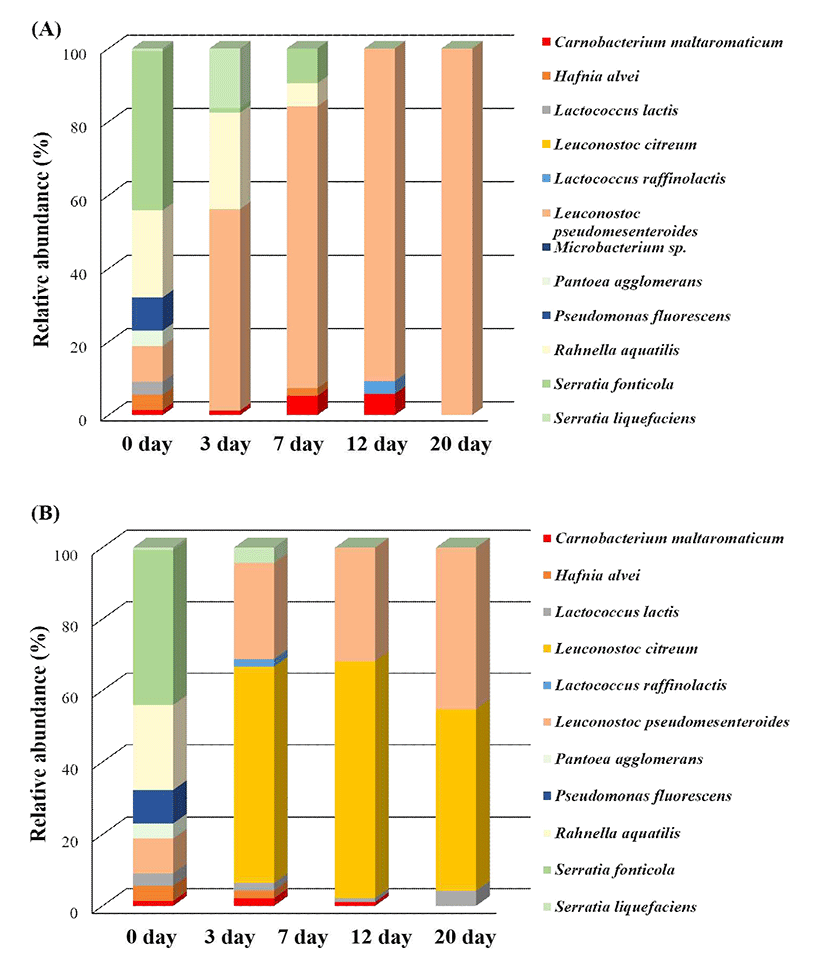
The storage of minimally processed PR at 15℃ was associated with increased relative abundances of L. citreum, L. pseudomesenteroides, and Lactobacillus lactis (Fig. 3B) and decreased relative abundances of P. fluorescens, R. aquatilis, and S. fonticola. The most abundant bacteria during the entire storage period at 15℃ were L. citreum and L. pseudomesenteroides, with relative abundances of 50.5 and 45.3%, respectively, at the end of storage. At day 0, L. citreum accounted for less than 1% of the community, but these bacteria dominated after day 1 with a relative abundance of 60.2%, which was followed by L. pseudomesenteroides (26.9%). At day 3, L. citreum was still present at the highest relative abundance (65.9%); it then decreased to 50.5% at day 7 but was still the predominant bacteria. L. pseudomesenteroides was the second most dominant bacteria, and its relative abundance gradually increased to 26.9% at day 2 and 31.9% at day 3. After 7 days of storage, the relative abundance of L. pseudomesenteroides was 45.3%, and its relative abundance was similar to that of L. citreum after the same storage period. During storage at 15℃, the relative abundances of S. fonticola, R. aquatilis, P. agglomerans, and P. fluorescens decreased, changing from 43.4, 23.8, 4.2, and 9.1%, respectively, at day 0 to 0% at day 1. L. lactis accounted for less than 5% of the community during storage at 15℃ but was consistently present under those conditions.
Storage results revealed that the abundance of spoilage-associated bacteria significantly increased, with an increase in TVCs in stored, minimally processed PR at 5 and 15℃, by the end of storage (Fig. 4A and 4B). TVCs increased by 1 log CFU/g and reached 7 log CFU/g during storage at 5℃, and the relative abundance of Leuconostoc spp. (especially L. pseudomesenteroides) increased from approximately 10% at the initial day to 100% at 20 days of storage, whereas that of Serratia spp. including S. fonticola and S. liquefaciens, decreased from approximately 40 to 0% over the same storage period. As TVCs increased from 6 log CFU/g on day 0 to 8 log CFU/g at day 7 of storage at 15℃, the relative abundance of Leuconostoc spp. including L. citreum and L. pseudomesenteroides increased from less than 10% to 90% at day 7 of storage.
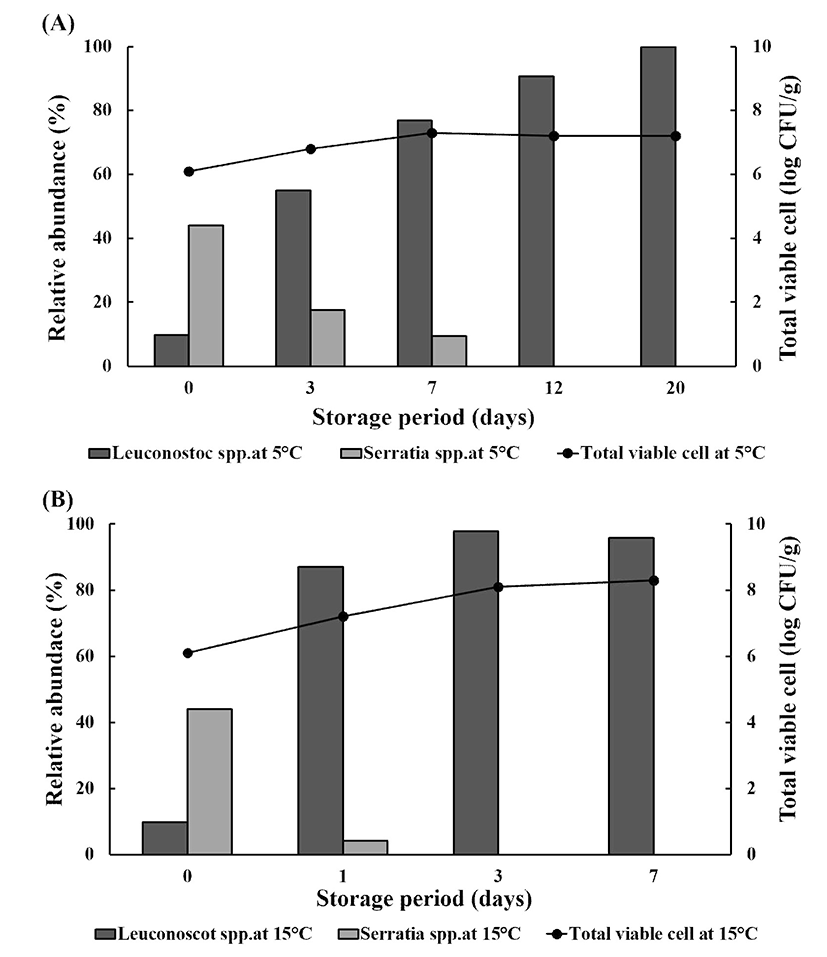
Discussion
Microbiological spoilage is one of the major reasons for shelf life failure for most packaged and refrigerated fresh-cut fruits and vegetables; this is followed by surface discoloration, off-aroma, and flavor changes. Microbial growth has been used as the main criterion to determine the shelf life of fresh-cut vegetables (24). Fresh PR is very sensitive to quality changes due to its respiration response (25). If PR is not properly treated (i.e. stored for too long at an inappropriate temperature), its flesh changes to a grayish-yellow or reddish-yellow color from white and it produces off-odors. The study of Park et al. (26) reported the relationship between the packaging method and deterioration of peeled PR under different storage temperatures. The pH value of peeled PR under vacuum packaging was also found to decrease from 6.0 to 5.1 after 5 days at 4℃ and 6.0 to 4.8 after 4 days of storage at 10℃. They also demonstrated that the hardness of peeled PR declined significantly faster at 10℃ (2 days storage) than at 5℃ (5 days storage) (25).
The microbial spoilage of root or tuber vegetables such as PR has been scarcely described, and no recent publications about this phenomenon are available. The aim of this study was to determine changes in microbial load following the processing of PR and to investigate changes in the initial dominant bacterial communities during storage that are established by processing. In this study, microbiological quality did not significantly change after each processing step. In minimally processed products, washing is a key step to remove dirt and cell exudate from harvested produce or to reduce the microbial population on food surfaces (26). The bacterial abundance in this study was unexpectedly higher, by approximately 1 log CFU/g, after the washing step and in the packaged end-product from both companies, compared to that in the raw PR. The average levels of TVCs, Enterobacteriaceae, and coliforms in the end-product of two different companies were 5-6 log CFU/g, and TVCs reached 7 log CFU/g in the end-product. For TVCs, the presence of greater than 5 log CFU/g in ready-to-eat food generally indicates poor storage conditions, poor handling practices, and the use of low-quality ingredients (27). Enterobacteriaceae and coliforms have also been used as indicator organisms to suggest poor hygiene or inadequate processing, processing failures, and the post-process contamination of foods (28). This is because almost all Enterobacteriaceae are killed by appropriate cleaning procedures. Although there are no guideline limits for minimally processed products in Korea, changes in the levels of these groups should be investigated during processing or storage to improve microbiological quality. The findings of 6 log CFU/g of Enterobacteriaceaeand 7 log CFU/g of coliforms in the end-products from both companies are in accordance with the results of Froder et al. (29), who found more than 5 log CFU/g of coliforms and Enterobacteriaceae in 60% of ready-to-eat salads. Sagoo et al. (30) also reported Enterobacteriaceae in all collected ready-to-eat salads from retail markets. This result suggests that the current processes of washing by dipping in or rinsing with running water did not reduce microorganism populations. This result suggests a potential risk for shelf-life failure and food safety and developing better washing techniques is therefore necessary to improve microbiological quality and extend shelf-lives.
The vacuum packaging and low temperature storage of end-products was also found to affect their microbiological quality. Since root vegetables such as PR and Panax ginseng Meyer consist of living tissues that respire, vacuum packaging decreases O2 content, increases CO2 content, and facilitates the establishment of psychrotrophic bacteria. These biological changes might trigger physiological deterioration, leading to color and texture changes and spoilage (31). TVCs in end-products stored at 5℃ increased by only 1 log CFU/g from its initial level before storage. The initial levels of fungi, Enterobacteriaceae, and coliforms also remained constant for 7 days but then decreased to less than 2 log CFU/g by the end of storage. These results agree with the results of Aksu et al. (32), who reported that vacuum packaging or modified atmosphere packaging (MAP) significantly prevents the growth of Enterobacteriaceae. Gram-negative bacteria are generally more sensitive to CO2 than gram-positive bacteria (33) because most gram-positive bacteria are facultative or strict anaerobes (34). Fungi are aerobic microorganisms, and oxygen depletion, which is commonly induced by vacuum packaging, could inhibit their growth; however, it is not lethal to these microorganisms (35,36). The levels of Enterobacteriaceae and coliforms were approximately 1 log CFU/g at the end of storage in this study. In contrast, 15℃ storage with vacuum packaging was associated with increased TVC levels during storage. After 3 days of storage at 15℃, TVC levels were higher compared to those in PR stored at 5℃. In contrast, Enterobacteriaceae and coliform levels decreased more rapidly at 15℃ than at 5℃, although their levels at the end of storage were similar for both storage temperatures. Long-term storage of PR at 5 and 15℃ had a positive effect on inhibiting the growth of microorganisms such as fungi, Enterobacteriaceae, and coliforms; in contrast, growth inhibition of TVCs failed at both 5 and 15℃. We hypothesized that the elevated TVC levels observed in PR stored at 5 and 15℃ might be associated with the survival of psychrophilic bacteria and not Enterobacteriaceae and coliforms, as the final levels of these bacteria decreased from 5-6 log CFU/g to 1 log CFU/g at the end of storage. This study analyzed dominant bacterial communities during processing and the change in bacterial composition after storage at 5 and 15℃.
We observed distinct characteristics and variation in the dominant bacterial communities after minimal processing, which was analyzed by MALDI-TOF MS. P. fluorescens and S. liquefaciens were dominant bacteria in the raw material prior to processing; in contrast, S. fonticola, R. aquatilis and L. pseudomesenteroides dominated the end-product. The relative abundance of P. fluorescens decreased, whereas S. fonticola increased, when raw material was shredded. The relative abundance of S. fonticola increased during washing, and L. pseudomesenteroides was only detected in minimally processed PR with vacuum packaging. These results indicate that predominant bacteria in minimally processed PR are associated with biochemical changes induced by processing steps such as shredding and washing; this might indicate that the processing of minimally processed products exerts a selective force on the composition of the bacterial community. Many studies have already investigated the effect of processing on the microbiological quality of minimally processed products (37,38) and a few studies have investigated changes in the bacterial composition following processing steps. Van Houdt et al. (39) reported that the bacteria isolated during the processing of fresh-cut vegetable were Vibrio diazotrophicus, Serratia plymuthica, and P. agglomerans and that the richness and abundance of the bacterial community in raw materials differed based on the processing step. Perez-Rodriguez et al. (40) showed that raw material sources, worker hygiene practices, and processing practices affected the composition of microflora in raw materials.
The growth of lactic acid bacteria (LAB) varies depending on the type of product that is packaged. The increased CO2concentration and O2 depletion caused by vacuum packaging are suitable for the growth of LAB. These changes can accelerate the spoilage of susceptible products such as lettuce, chicory leaves, and carrots by LAB (14,41,42). This study found that dominant bacterial communities change over the entire period of cold storage. PR stored at 5 and 15℃ was dominated by Enterobacteriaceae (i.e. R. aquatilis, S. liquefaciens, and S. fonticola) on the first day of storage, whereas LAB such as L. pseudomesenteroides and L. citreum, representative spoilage-associated bacteria, dominated at the late stages of storage. The dominant bacterial communities during storage slightly differed with the two storage temperatures. At the end of storage, the bacterial community at 5℃ was dominated by L. pseudomesenteroides; in contrast, L. citreum and L. pseudomesenteroides predominated at 15℃.
When the deterioration of products occurs as appearance defects and faults related to flavor, this manifestation is generally attributed to the dominant bacteria, usually referred to as specific spoilage organisms. In our study, off-flavors and off-tastes such as acidity appeared after 7 days of storage at 5℃ and 2 days at 15℃ (data not shown). Park et al. (25) reported that the sensory attributes of peeled PR stored at 4℃ decreased after 7 days, whereas those of peeled PR at 10 and 20℃ decreased after 2 days of storage. The flavor and texture scores of peeled PR stored at 20℃ remarkably decreased to 1.9 from 7.9 after 2 days. However, the reason for this observation is not clear. We suggest that changes in the flavor and taste of minimally processed PR might be attributed to the development of Leuconostoc spp., as the relative abundance of L. pseudomesenteroides increased after 7 days at 5℃ and that of L. citreum and L. pseudomesenteroides increased after only 2 days at 15℃. LAB is useful for the production of fermented foods such as soy sauce and cheese (43). Although the importance of LAB is widely recognized, acidic off-flavors, off-odors, decrease in pH levels, milky exudates, gas production, swelling of packs, discolorations, and greenish colors can generally be observed as a result of these bacteria, and especially Leuconostoc activity (44). In our study, L. pseudomesenteroides was the predominant bacteria in minimally processed PR during storage at 5℃, and was also the major species at 15℃. L. pseudomesenteroides, belonging to LAB, is associated with the fermentation of vegetables and other products (45). L. pseudomesenteroides produces CO2 and acids, which rapidly lower the pH and inhibit the development of other psychrotrophic organisms (44).
Pseudomonas, representative of spoilage bacteria, was not isolated during storage. Under vacuum conditions, the microbial population is determined by environmental conditions such as temperature, relative humidity, and partial O2 and CO2concentration (46). In general, the atmospheric conditions under vacuum packaging change during storage, which results in decreases in the oxygen concentration (to 1%) and an increases in the CO2 concentration (to 20-25%) (47). Goulas et al. (48) found that increasing the CO2concentration inhibits the growth of aerobes such as Pseudomonas. The results observed in this study are in agreement with those results (48). In addition, Pseudomonas growth is inhibited by pH levels less than 5.4 (49). This result also suggests that the predominance of L. pseudomesenteroides during storage under low temperatures can induce a reduction in pH levels, and that this biological change might suppress the growth of other psychrophilic spoilage bacteria such as Pseudomonas, Serratia, and Rahnella.
In this study, TVC levels during storage were associated with the abundance of Leuconostoc spp. The relative abundance of Leuconostocspp. and Serratia spp. at the end of storage at 5 and 15℃ indicated that this microbial group dominate in terms of final counts in the minimally processed PR stored under vacuum conditions because TVC levels were constantly maintained at 7 log CFU/g during storage at 5℃ or increased to 8 log CFU/g after storage at 15℃. The results of this study suggest that the quality loss during storage under low temperature might be associated with an increase in the relative abundance of Leuconostoc spp. These bacteria were previously reported to be the dominant microbes in spoiled products after MAP or vacuum packaging (13,14). Concentrations of LAB exceeding 6 log CFU/g with vacuum packaging are particularly important for their high potential for food deterioration and food safety (12). In this study, the identification of microbial communities can provide insights into the spoilage progress by microorganisms. The relationships between deterioration and spoilage-related bacteria under different storage conditions, based on appropriate molecular approaches, should be evaluated to broaden our knowledge of bacterial mechanisms related to food spoilage in vacuum packaged products during storage.
Conclusion
The relative abundance of bacterial communities differed based on environmental changes. Specifically, dominant bacterial communities responded differently to various environmental conditions such as the processing step, storage temperature, and period. The relative abundance of spoilage-associated bacteria remarkably increased during storage. L. pseudomesenteroides was the dominant bacteria representative of minimally processed PR stored at 5℃, whereas L. citreum and L. pseudomesenteroides also comprised the main bacteria groups in samples stored at 15℃. Increases in the relative abundances of Leuconostoc spp. are the likely cause of the appearance of off-flavors and off-tastes in samples during long-term storage at low temperatures. L. pseudomesenteroides thus seems to be a key player in PR spoilage throughout the entire storage period under vacuum packaging and in cold storage as it was found in samples stored at either 5 or 15℃ and was isolated at both early and late stages of storage. The knowledge of dominant bacterial communities might provide new approaches to prolong the freshness of minimally processed tuber and root vegetables. Studying the molecular response of spoilage-related bacteria to different packaging and storage conditions will be fundamental to improve the freshness of tuber and root vegetables during storage.