Introduction
A demand for high quality but safe food has been on the rise for both food industry and consumers worldwide. Although several intervention strategies have been introduced and implemented, the number of foodborne outbreaks is either increasing and/or steady, depending on the causative foodborne pathogens (1). Salmonella, along with Escherichia coli O157:H7, has been the most prevalent foodborne pathogen (2-3). Approximately 1.4 million cases of Salmonella infections are reported in the United States every year, and the annual cost for medical treatment and lost productivity has been estimated to be 0.5 to 2.3 billion dollars (1). The general symptoms of Salmonella infections are characterized by fever, headache, abdominal pain, diarrhea or constipation, vomiting, and nausea (4-5). So far, out of more than 2,500 serotypes of Salmonella, S. Typhimurium, S. Enteritidis, S. Newport, S. Javiana, and S. Heidelberg are the most frequently reported (3,6,7). Salmonella outbreaks have mainly been associated with the consumption of meat, poultry, milk, and dairy products contaminated with Salmonella. In the past two decades, Salmonella outbreaks have been increasing associated with minimally processed fruits and vegetables, such as seed sprouts, unpasteurized fruit juices, mangoes, tomatoes, and spinach (3,7,8).
Considerable studies have been performed for monitoring and controlling Salmonella contamination by using PCR, ELISA, and various biosensor methods (9-10). Since the last two decades, biosensor method has been recognized as an on-site applicable rapid detection method for food industry and its consumers (10-11). Biosensor is defined as an analytical device that converts recognition of a target analyte into a measurable signal by using a physicochemical transducer (12). Our research group has already developed a gold biosensor combined with a light microscopic imaging system (LMIS), for the detection of Listeria monocytogenes and E. coli O157:H7 in food (13-15). In the detection system, the specific and reactive antibody is immobilized on the gold sensor platform for the specific recognition of the target pathogens. When the antibody-immobilized gold sensor is placed on the surface of a food, specific binding of target pathogens to the antibody occurs on the gold sensor. Finally, the bound target pathogen is visualized and enumerated by a light microscope connected with a charge-coupled device (CCD) camera (13). Using the same principle and methodologies, the current system is expected to detect Salmonella in food as a on-site applicable method.
There are several bio-recognition elements available including tissues, cells, enzymes, antibodies, and nucleic acids. Among them, the antibody has been chosen most popularly due to its excellent reactivity (sensitivity) and specificity (16-17). Since antibodies are produced by lymphocytes as defense mechanism in response to antigens in vertebrates, they show excellent reactivity and specificity against their targets. The overall goal of our study was to develop a rapid, on-site applicable gold biosensor method combined with LMIS for Salmonella detection. In order to construct the biosensor method, anti-Salmonella polyclonal antibodies were produced and purified from a rabbit. The characteristics of purified antibodies including reactivity and specificity, and capturing capability against Salmonella were investigated as a bio-recognition element in this study.
Materials and Methods
All the foodborne pathogens listed in Table 1, except for bacteria ending with ATCC, were obtained from the Food Microbiology Laboratory at Auburn University Auburn, AL, USA. The other foodborne pathogens with ATCC label were obtained from American Type Culture Collection (ATCC, Rockville, MD, USA). Each bacterial strain was cultivated in 20 mL of Tryptic Soy Broth (TSB, Difco Laboratories, Sparks, MD, USA) while L. monocytogenes was cultivated in TSB with 0.6% yeast extract. After incubation at 37℃ at 200 rpm for 16 h, each culture was washed three times with phosphate buffered saline (PBS, pH 7.2, Sigma-Aldrich Co., St. Louis, MO, USA) by centrifugation at 5,000 ×g for 5 min. The collected bacterial cells were re-suspended in PBS and the bacterial concentration was adjusted to 108 CFU/mL using a pre-constructed standard curve, determined by optical density at 640 nm. Salmonella mixture was prepared by mixing equal amounts of S. Typhimurium and S. Enteritidis.
A New Zealand white rabbit (approximately 3 kg) was used for the production of pAbs against Salmonella. The purification of pAbs was performed following a modified Kohler and Milstein’s method (1975). For the immunization of the rabbit, Salmonella mixture (108 cells), inactivated with 1% formalin, was emulsified with Ribi’s adjuvant system at 285 g/mL. The rabbit was immunized with 1 mL of the adjuvant emulsion through an intradermal injection of 50 μL into each of eight sites on the back, close to the spinal cord, and 300 μL aliquot intramuscular into each hind leg. The same immunization procedure with the adjuvant emulsion was performed to boost the antibody production in the rabbit at 4 week intervals after the initial immunization. Seven days later, the rabbit blood containing anti-Salmonella pAbs was collected from the central ear artery and centrifuged at 5,000 ×g for 20 min at 4℃. Solid ammonium sulfate was added slowly into 1 mL of the collected anti-Salmonella rabbit serum until a final concentration of 0.5 g/mL. After placing on ice for 5 min, the serum was centrifuged at 5,000 ×g for 5 min at 4℃ and the precipitate was washed twice with 1 mL of saturated ammonium sulfate. The precipitate was then suspended in PBS and dialyzed in a 20 mM sodium phosphate buffer (pH 7.0) at 4℃ overnight. The suspension containing anti-Salmonella pAbs was applied to protein A affinity column (Sigma-Aldrich Co., St. Louis, MO, USA) and eluted with 0.1 M citric acid (pH 3.45). After adjusting pH of the eluates with 2 M Tris (pH 9), the protein content of each fraction was estimated by measuring adsorption at 280 nm. Finally, all the eluates were dialyzed with PBS at 4℃ overnight, and the protein content of purified anti-Salmonella pAbs was determined by Bradford method.
Salmonella mixture (S. Typhimurium and S. Enteritidis) and forty foodborne pathogens (20 Salmonella serotypes and 20 non-Salmonella strains) were used for the investigation of the reactivity and specificity of anti-Salmonella pAbs, respectively. A 96-well polystyrene assay plate (Costar, Cambridge, MA, USA) was coated with 100 μL of Salmonella mixture for the reactivity test and each bacterial suspension (108 CFU/mL) for specificity test at 37℃ for 1 h. After washing three times with 200 mL PBS containing 0.1% Tween-20 and 0.02% sodium azide (PBST), the plate was blocked with 200 μL of 1% bovine serum albumin (BSA, Equitech-Bio Inc., Kerrville, TX, USA) for 1 h at 22℃, followed by washing with 200 mL PBST. An aliquot of 100 μL of purified anti-Salmonella pAbs was added and the plate was incubated at 22℃ for 2 h, prior to washing with PBST. Commercial anti-Salmonella pAbs (Abcam Co., Cambridge, MA, USA) were used for the comparison of reactivity with that of purified anti-Salmonella pAbs. An aliquot of 100 μL of alkaline phosphatase-conjugated goat anti-rabbit antibodies (Sigma-Aldrich Co.) was added to each well for incubation at 22℃ for 1 h. Finally, 100 μL of p-nitrophenyl phosphate (p-npp, Sigma-Aldrich Co.) in 10 mM diethanolamine buffer (pH 9.5) was added to the wells for color development. Finally, the absorbance at 405 nm was measured at 0 min and 15 min using a microplate reader (Thermo Labsystems Inc., Helsinki, Finland).
A glass sensor platform was prepared by cutting a microscopic cover glass (0.17 mm thickness) into 5×5 mm with micro-dicing saw (MPE Inc., Grass Valley, CA, USA). After cutting, it was ultrasonically cleaned with an acetone solution, and rinsed with alcohol and deionized (DI) water. The cleaned sensor was sputtered with 140 nm gold by using a Pelco SC-6 sputter coater (Ted Pella Inc., Redding, CA, USA). For antibody immobilization, 100 μL of purified anti-Salmonella pAbs were added on the gold sensor surface for incubation at 37℃ for 1 h. After washing three times with PBS, the pAbs-immobilized gold sensor was blocked with 100 μL of 1% BSA at 22℃ for 1 h. Finally, the sensor was incubated with Salmonella mixture at 37℃ for 1 h and washed four times with DI water, prior to SEM observation (S-4800, Hitachi Ltd., Tokyo, Japan).
All experiments were performed at least in triplicates and the data are expressed as mean±SD. Student’s paired t-test, for two groups, and one-way analysis of variance (ANOVA), for more than two groups, were used to compare the means by using GraphPad and InStat V.3 software (GraphPad Co., San Diego, CA, USA). Differences were considered to be statistically significant at p<0.05.
Results and Discussion
Unlike the preparation of monoclonal antibodies (mAbs), the preparation of pAbs is less complicated since it does not require an immortal hybridoma (18-19). Anti-Salmonella pAbs were produced from a rabbit and purified by saturated ammonium sulfate precipitation and protein A affinity column. The final antibody concentration was determined to be 2.0 mg/mL, which was greater than that of commercial anti-Salmonella pAbs (1.0 mg/mL).
Reactivity of purified anti-Salmonella pAbs was tested and compared with that of commercial ones by indirect ELISA (Fig. 1). Since the original concentration of purified anti-Salmonella pAbs was two-fold greater than that of commercial anti-Salmonella pAbs, its concentration was adjusted with that of commercial ones to enable the direct comparison between both antibodies. The reactivity of purified anti-Salmonella pAbs was greater than those of commercial anti-Salmonella pAbs over the entire range of pAbs concentration, although there were no significant differences between the two groups at the same dilution of anti-Salmonella pAbs (p>0.05). Higher the antibody concentration, less differences of absorbance was observed for both anti-Salmonella pAbs. However, the purified anti-Salmonella pAbs obviously had greater binding efficiency against Salmonella mixture at relatively lower concentration of pAbs, ranging from 1/3,200 to 1/6,400 dilution of the original concentration of pAbs (1.0 mg/mL). The greater binding efficiency of purified anti-Salmonella pAbs over commercial pAbs can presumably be attributed to targeting S. Typhimurium and S. Enteritidis and relatively delicate purification procedures. Based on our results, the concentration of purified anti-Salmonella pAbs was determined to be 0.00125 mg/mL (1,600 times dilution of original concentration of purified anti-Salmonella pAbs) considering both reactivity and economic aspects. Finally, this study confirmed that the purified anti-Salmonella pAbs had a competitive reactivity over commercial anti-Salmonella pAbs.
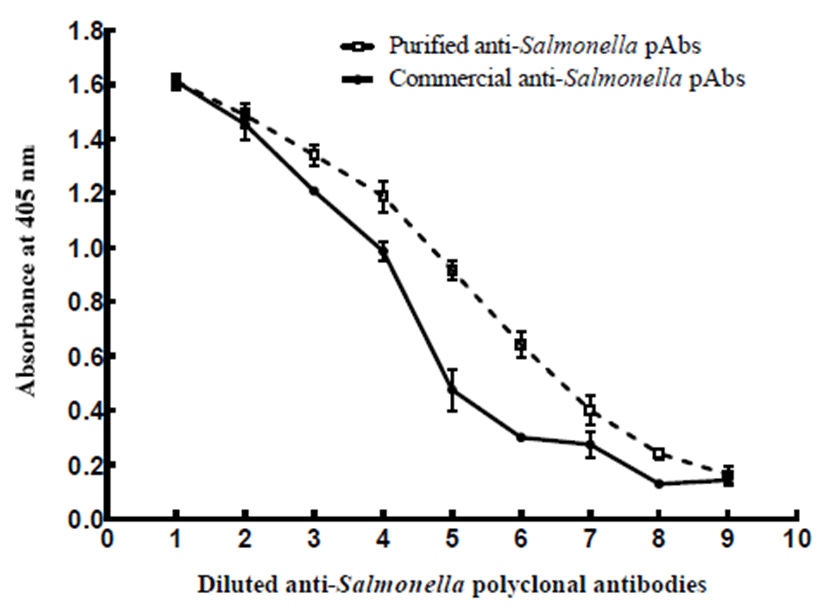
Besides reactivity, the other desired property, for the antibody to be useful as a bio-recognition element, is its specificity against all pathogenic Salmonella serotypes. So far, 20 most frequent serotypes have been held responsible for approximately 70% of Salmonella outbreaks (approximately 13% unknown serotypes and 15% other serotypes) (7). As shown in Table 1, all Salmonella serotypes, except S. Diarizonae, showed significantly higher binding efficiencies with purified anti-Salmonella pAbs (p<0.05). Among the 20 Salmonella serotypes, purified anti-Salmonella pAbs showed the best binding reactivity with S. Typhimurium and S. Enteritidis, which exhibited maximum color change during incubation. However, the purified anti-Salmonella pAbs showed no cross-reactivity against other exclusive foodborne pathogens including major strains of Bacillus, Campylobacter, E. coli/E. coli O157:H7, Listeria, Shigella, Staphylococcus, Vibrio, and Yersinia. Their absorbance changes were obviously lower than those of Salmonella serotypes, except for S. Diarizonae.
Based on the recent annual report of Salmonella outbreaks, there were 45,735 cases recognized by CDC in 2013 (7). Among them, S. Typhimurium and S. Enteritidis accounted for 12.8% and 15.1%, respectively. Our purified antibodies showed the best reactivity with these two major serotypes of Salmonella. Furthermore, our pAbs also showed binding efficiency with some of the recent top 20 major serotypes of Salmonella including S. Newport, S. Javiana, S. Heidelberg, S. Montevideo, and S. Typhi. Overall, the purified anti-Salmonella pAbs showed an excellent specificity against pathogenic Salmonella.
For the efficient performance of purified anti-Salmonella pAbs, the antibodies need to bind with Salmonella after their immobilization on the gold sensor surface (15). If the purified antibodies are immobilized on gold sensor properly, their binding with Salmonella will be increased so that numerous Salmonella can bind on the antibody-immobilized sensor surface. As seen in Fig. 2B and 2C, there were numerous bindings of Salmonella observed, corresponding with the increase in Salmonella concentration. Meanwhile, there are a few non-specific binding of Salmonella on the sensor surface devoid of antibody, but they are very weak (p<0.05). Capture capacity of the pAbs against Salmonella could be enhanced by using various immobilization methods, such as a covalent attachment with cross-linker and the application of protein A, G, and other derivatives on the sensor platforms (13,14,20,21). However, such studies will be reserved for the future, and our focus for this report will remain on developing new bio-recognition element for Salmonella detection with excellent reactivity and specificity.
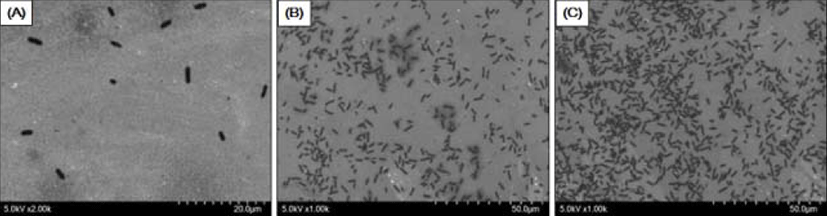
In summary, anti-Salmonella pAbs were successfully isolated and purified from a rabbit and demonstrated competitive reactivity over commercial anti-Salmonella pAbs. In addition, it showed excellent specificity against pathogenic Salmonella serotypes and sufficient binding capability with Salmonella on the gold sensor. Therefore, the purified anti-Salmonella pAbs could be a novel bio-recognition element for application in a gold biosensor combined with LMIS for Salmonella monitoring.
요 약
살모넬라는 주요한 식중독균의 하나로 미생물학적 식품 품질을 보증하기 위해 신속·정확하게 현장에서 관리되고 모니터링되어야 한다. 본 연구팀에서는 광학 현미경 기반 의 이미징 시스템과 바이오센서가 결합된 모니터링 시스템 을 개발하였고, 이를 살모넬라에 적용하기 위해서는 바이 오센서에 적용되는 생물학적 수용체의 개발이 절대적으로 필요한 실정이다. 따라서 본 연구에서는 살모넬라에 반응 하는 특이적인 anti-Salmonella 다중클론 항체를 토끼로부 터 성공적으로 생산·정제하였으며, 최종 항체의 농도는 2 mg/mL로 결정되었다. 또한, 정제된 anti-Salmonella 다중클 론 항체의 역가가 상업용 anti-Salmonella 다중클론 항체보 다 전 항체 농도 범위에서 우수함을 알 수 있었다. 정제된 항체 특이성 검토를 위해, 정제된 anti-Salmonella 다중클론 항체를 20 종의 살모넬라 serotypes과 20 종의 non-Salmonella strains과 반응시킨 결과, 20 종의 non-Salmonella strains과 는 특이성을 보여주지 않은 반면 19 종의 살모넬라 serotypes와 유의적으로 높은 반응성으로 보여 주었다. 최 종적으로, anti-Salmonella 다중클론 항체가 고정화된 골드 센서를 살모넬라 현탁액에 노출시켰을 때, 수 백여 개의 살모넬라가 센서 표면에 capture되어 있음을 확인할 수 있 었다. 따라서, 본 연구를 통해 생산·정제된 anti-Salmonella 다중클론 항체는 새로운 생물학적 수용체로서 충분한 역가 와 특이성 그리고 센서에 적용 시 살모넬라와의 높은 결합 력을 보여주었으므로 광학현미경 기반 이미징 시스템을 활용한 바이오센서 모니터링 시스템에 새로운 생물학적 수용체로 적용될 수 있으리라 판단된다.