1. Introduction
Advances in medicine have indeed extended human life expectancy; however, aging remains a factor that diminishes metabolic and immune functions while also affecting the central nervous system, contributing to various chronic diseases (Gentile et al., 2023). Globally, the aging population is on the rise, paralleled by an increase in the prevalence of neurodegenerative diseases (ND), which impose significant burdens on both society and the medical system. By 2050, it is estimated that approximately 115 million people will be affected by ND (Enogieru et al., 2018; Feng et al., 2023; Gentile et al., 2023). ND, encompassing Alzheimer’s disease, Parkinson’s disease, and Huntington’s disease, manifests as a gradual decline in neurons, impacting cognitive and motor functions (Enogieru et al., 2018; Uddin et al., 2020).
Several studies have implicated oxidative stress as the principal pathogenic mechanism underlying ND. This oxidative stress arises from an imbalance between reactive oxygen species (ROS), such as the superoxide radical (O2•-), hydrogen peroxide (H2O2), hydroxyl radical (•OH), and singlet oxygen (1O2), generated during in vivo metabolic processes, and the body’s antioxidant system responsible for scavenging them (Kowalczyk et al., 2021).
The brain, consuming 20% of the body’s oxygen, is particularly susceptible to ROS due to its active metabolism. Its vulnerability to oxidative stress stems from a deficiency in endogenous antioxidants such as glutathione (GSH) (Kim et al., 2015). Excessive ROS production coupled with impaired antioxidant system function leads to protein denaturation, DNA damage, and enzyme inactivation, ultimately resulting in neuronal cell death (Uddin et al., 2020). Additionally, the highly reactive nature of ROS, compounded by structural instability, triggers reactions with unsaturated fatty acids in the brain, leading to structural and functional damage to cell membranes and the generation of reactive molecules such as malondialdehyde (MDA), acrolein, and 4-hydroxy-2-noneal (HNE). These compounds promote cell damage and the development of ND (Kowalczyk et al., 2021; Taso et al., 2019).
Controlling oxidative stress has emerged as a crucial strategy for preventing ND, prompting active research to identify antioxidants from plant sources deemed safe for human consumption (Enogieru et al., 2018; Jeong et al., 2021).
A. yomena, a plant of the Asteraceae family, has a long history of medicinal and edible use and is widely distributed in Asia. It contains various phytochemicals, including chlorogenic acid, rutin, caffeic acid, esculetin, and isoquercitrin (Kim et al., 2018; Kim et al., 2020; Park et al., 2021). A. yomena, which contains these bioactive compounds, has been associated with anti-obesity, skin protection, anti-inflammatory, and antibacterial effects in in vitro studies, while in vivo and ex vivo studies in mice have reported anti-asthmatic and anticoagulant properties (Kim et al., 2018; Seo, 2020). Despite these findings, research on the neuroprotective effects of A. yomena ethanol extract is limited. Therefore, this study aims to investigate the in vitro antioxidant activity and neuronal protective effects of A. yomena 60% ethanol extract and analyze potential physiological activity candidates within A. yomena.
2. Materials and methods
The reagents utilized in this study, including Folin & Ciocalteu’s phenol reagent, Na2CO3, gallic acid, NaOH, diethylene glycol, rutin, sodium phosphate buffer, potassium persulfate, 2,2′-azino-bis (3-ethyl benzothiazoline-6-sulfonic acid) (ABTS), 1,1-diphenyl 2-picrylhydrazyl (DPPH), trichloroacetic acid (TCA), thiobarbituric acid (TBA), ascorbic acid, catechin, Tris HCl buffer, 2′,7’-dichlorofluorescin diacetate (DCF-DA), 3-(4,5-dimethyl-thiazol-2-yl)-2,5-diphenyl tetrazolium bromide (MTT), Dulbecco’s Modified Eagle Medium (DMEM), calf serum (CS), fetal bovine serum (FBS), penicillin, streptomycin, H2O2, and dimethyl sulfoxide (DMSO), were procured from Sigma-Aldrich Chemical Co. (St. Louis, MO, USA). All other solvents and reagents used were of Grade 1 or higher quality.
For this test, fresh leaves of A. yomena cultivated in Gurye, Jeollanam Province, were procured from Gurye Local Foods (Gurye, Korea) in May 2023. The leaves were thoroughly washed, freeze-dried, and ground before use. The ground samples were blended with ethanol solutions of varying concentrations: 0%, 20%, 40%, 60%, 80%, and 95%, and subjected to reflux extraction at 40°C for 2 h. Subsequently, the extracts were filtered using No. 2 filter paper (Whatman PLC, Kent, UK). The filtrates were concentrated employing a rotary vacuum concentrator (N-N Series, Eyela Co., Tokyo, Japan), freeze-dried, and stored at −20°C until further analysis. The yield of the 60% ethanol extract was determined to be 22.38%.
For the quantification of total phenolic compounds, the sample was combined with deionized water and Folin-Ciocalteu’s phenol reagent, and the mixture was allowed to react for 5 min at room temperature (20-25°C). Following the addition of 7% Na2CO3, the volume was adjusted to 25 mL using deionized water, and the reaction continued at room temperature for 2 h. Subsequently, the absorbance was measured at 760 nm using a spectrophotometer (UV-1800, Shimadzu, Kyoto, Japan), and the results were expressed as gallic acid equivalent (mg of GAE/g) based on a calibration curve (Abeysinghe et al., 2007).
To determine the total flavonoid content, the sample was mixed with diethylene glycol and 1 N NaOH and allowed to react at 30°C for 1 h. The absorbance was then measured at 420 nm using the same spectrophotometer (UV-1800, Shimadzu). The flavonoid content was calculated as rutin equivalent (mg of RE/g) using the absorbance values and a calibration curve established with rutin (Abeysinghe et al., 2007).
For the assessment of ABTS radical scavenging activity, a solution of 100 mM sodium phosphate buffer (pH 7.4) containing 150 mM NaCl was combined with 2.45 mM ABTS and 1.0 mM 2,2′-azobis (2-amidinopropane) dihydrochloride (AAPH) and allowed to react in a 68°C thermostatic water bath for 30 min. After cooling the ABTS solution to room temperature (20-25°C) for 10 min, it was filtered through a 0.45 μm filter and left to stand for 24 h before use. The prepared ABTS solution was adjusted to achieve an absorbance value of 0.70±0.02 at 734 nm and then mixed with the sample, reacting at 37°C for 10 min. Radical scavenging activity was determined by measuring the absorbance at 734 nm using a UV-1800 spectrophotometer (Shimadzu) (Floegel et al., 2011).
To evaluate DPPH radical scavenging activity, a 0.1 mM DPPH solution was prepared using 80% MeOH and then diluted to achieve an absorbance value of 1.00±0.02 at 517 nm. Upon addition of the DPPH solution to the sample, the mixture was allowed to react in a dark room for 30 min, after which the absorbance was measured at 517 nm using the UV-1800 spectrophotometer (Shimadzu) (Floegel et al., 2011).
This experiment subjected brain tissues, rich in unsaturated fatty acids, to oxidative stress to induce lipid peroxidation and evaluated the sample’s inhibitory effect by assessing the formation of red MDA-TBA adducts. Four-week-old male Institute of Cancer Research mice were procured from Samtako (Osan, Korea) for lipid peroxide inhibitory activity assessment. They were acclimatized for 1 week under controlled conditions of constant temperature (22±2°C) and humidity (50-55%), with a 12-h light/dark cycle. Subsequently, the brain tissue was extracted and homogenized using 20 mM Tris HCl buffer (pH 7.4), in a ratio of 10 times the tissue weight. The homogenate underwent centrifugation at 4°C and 12,000 ×g for 15 min to obtain pellets, tissue debris, and supernatant used for testing. The brain tissue supernatant was mixed with 10 μM FeSO4 and 0.1 mM ascorbic acid, and the mixture was allowed to react at 37°C for 1 h. Subsequently, 30% TCA and 1% TBA were added, and the reaction was carried out in an 80°C thermostatic water bath for 20 min. The absorbance of the resulting supernatant, obtained post-centrifugation, was measured at 532 nm using a UV-1800 spectrophotometer (Shimadzu) (Chang et al., 2001). This animal study was conducted with the approval of the Animal Ethics Review Committee of Gyeongsang National University (Approval Number: GNU-220429-M0042, Approval Date: 2022.04.29).
The Histology Laboratory at Gyeongsang National University College of Veterinary Medicine acquired HT-22 cells, a mouse hippocampal cell line, in October 2017. These cells were cultured in DMEM supplemented with 10% CS, 50 units/mL penicillin, and 100 μg/mL streptomycin under conditions of 37°C and 5% CO2 and were subsequently utilized for the experiment.
SK-N-MC cells, a human neuroblastoma cell line, were procured from the Korea Cell Line Bank in Seoul, Korea. These cells were cultured in DMEM supplemented with 10% FBS, 50 units/mL penicillin, and 100 μg/mL streptomycin under conditions of 37°C and 5% CO2 and were employed in the experiment.
HT-22 and SK-N-MC cells were seeded into a 96-well plate at a concentration of 1×104 cells/well to assess the viability of nerve cells. Following 24 h of incubation, the sample and positive control underwent processing and were cultured for an additional 24 h. Subsequently, they were exposed to 200 μM H2O2 and cultured for 3 h, after which they were treated with the MTT reagent and incubated at 37°C for 3 h. The culture medium was then aspirated, and DMSO was added. Absorbance was measured at 570 nm (determination wavelength) and 655 nm (reference wavelength) using a microplate reader (Epoch 2, BioTek Instruments Inc., Winooski, VT, USA) (Heo et al., 2001).
HT-22 and SK-N-MC cells were plated onto a black 96-well plate at a density of 1×104 cells/well to assess oxidative stress levels within neurons and incubated for 24 h. The sample and positive control underwent treatment and were cultured for an additional 24 h. Subsequently, they were exposed to 200 μM H2O2, cultured for 3 h, then treated with DCF-DA reagent, and incubated for 50 min. Fluorescence intensity was measured at 485 nm (excitation wavelength) and 535 nm (emission wavelength) using a fluorometer (Infinite F200, TECAN, Mannedorf, Switzerland) (Heo et al., 2001).
The bioactive substances present in the samples were analyzed using high-performance liquid chromatography (HPLC) with an Ultimate 3000 series system (Dionex, Sunnyvale, CA, USA). A YMC-Triart C18 column (250×4.6 mm, 5 μm, YMC Korea, Seongnam, Korea) was employed for separation. The samples were dissolved in 50% MeOH, filtered through a 0.45 μm filter, and 20 μL was injected into the system. The mobile phase consisted of distilled water (A) containing 0.1% formic acid and acetonitrile (B) containing 0.1% formic acid with a gradient elution profile: 0% B (0-2 min), 0%-50% B (2-22 min), 50-100% B (22-26 min), 100% B (26-28 min), and 100-0% B (28-30 min). The flow rate was set at 1 mL/min, and a diode array detector was used to monitor absorbance at 254 nm. The UV spectra of the sample were compared with those of standard materials to confirm similarity.
For quantitative analysis of the identified bioactive substances, standard substances were diluted stepwise and analyzed under the same conditions as the samples. Subsequently, a calibration curve was constructed using the chromatogram peak areas of the standard substances to determine concentrations relative to the curve.
The significance of the average value across all tests was validated using the Statistical Analysis System (SAS, SAS Institute Inc., Cray, NC, USA). Analysis of variance was conducted, and significant differences among each sample were assessed at the 5% level using Duncan’s multiple range test.
3. Results and discussion
Total phenolic and flavonoid contents, as well as ABTS and DPPH radical scavenging activities, were assessed to compare the antioxidant properties of 0%, 20%, 40%, 60%, 80%, and 95% ethanol extracts of A. yomena (Fig. 1). The 60% ethanol extract exhibited notably superior antioxidative activity compared to other extracts, showing values of 91.54 mg of GAE/g, 224.18 mg of RE/g, 49.66%, and 27.68%, respectively. Consequently, subsequent analyses were carried out using the 60% ethanol extract of A. yomena.
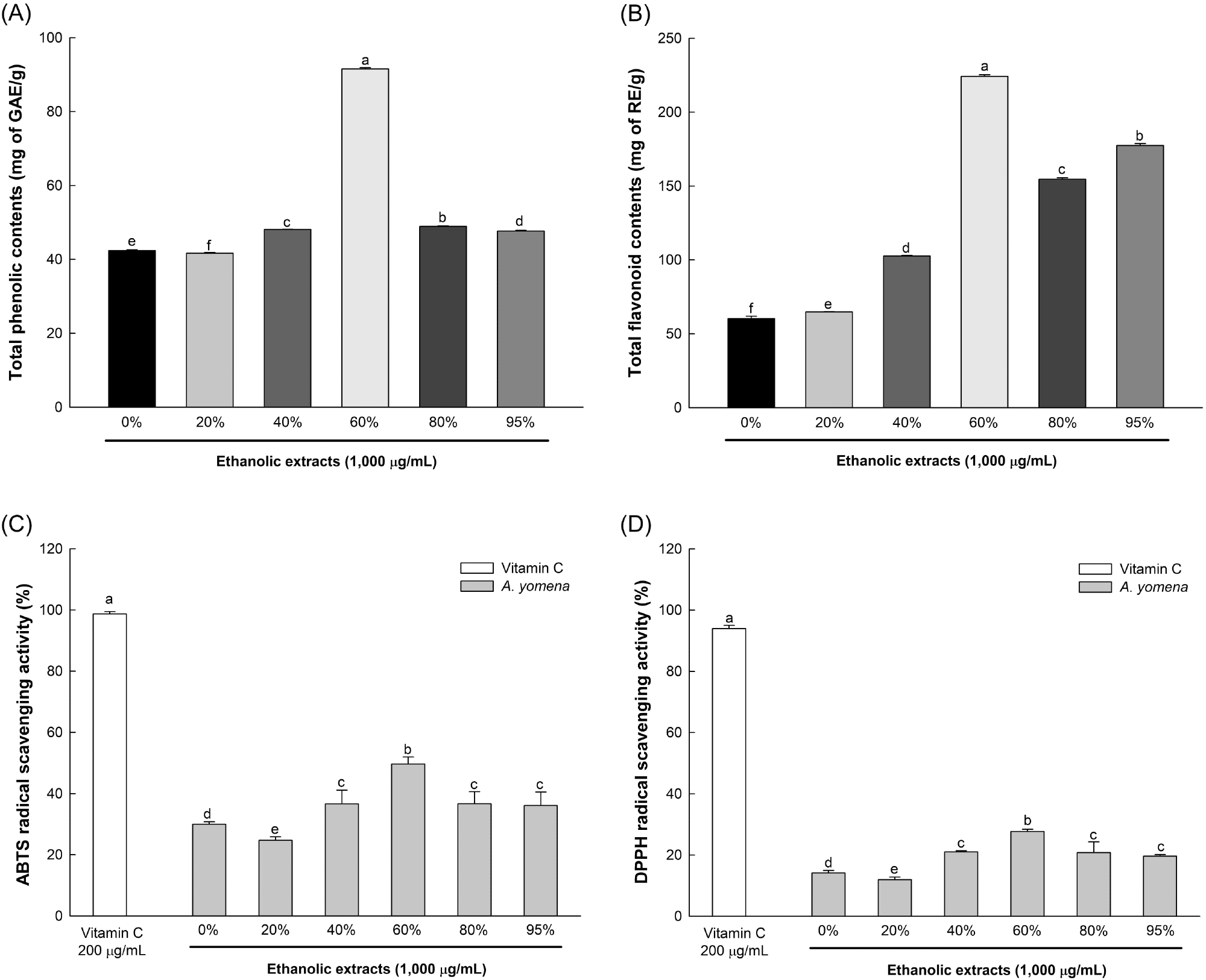
Further evaluation of the ABTS and DPPH radical scavenging activities of the 60% ethanol extract revealed IC50 values of 1,640.30 μg/mL and 2,655.10 μg/mL, respectively, with a lipid peroxide production suppression activity of 79.63% at 500 μg/mL (Fig. 2).
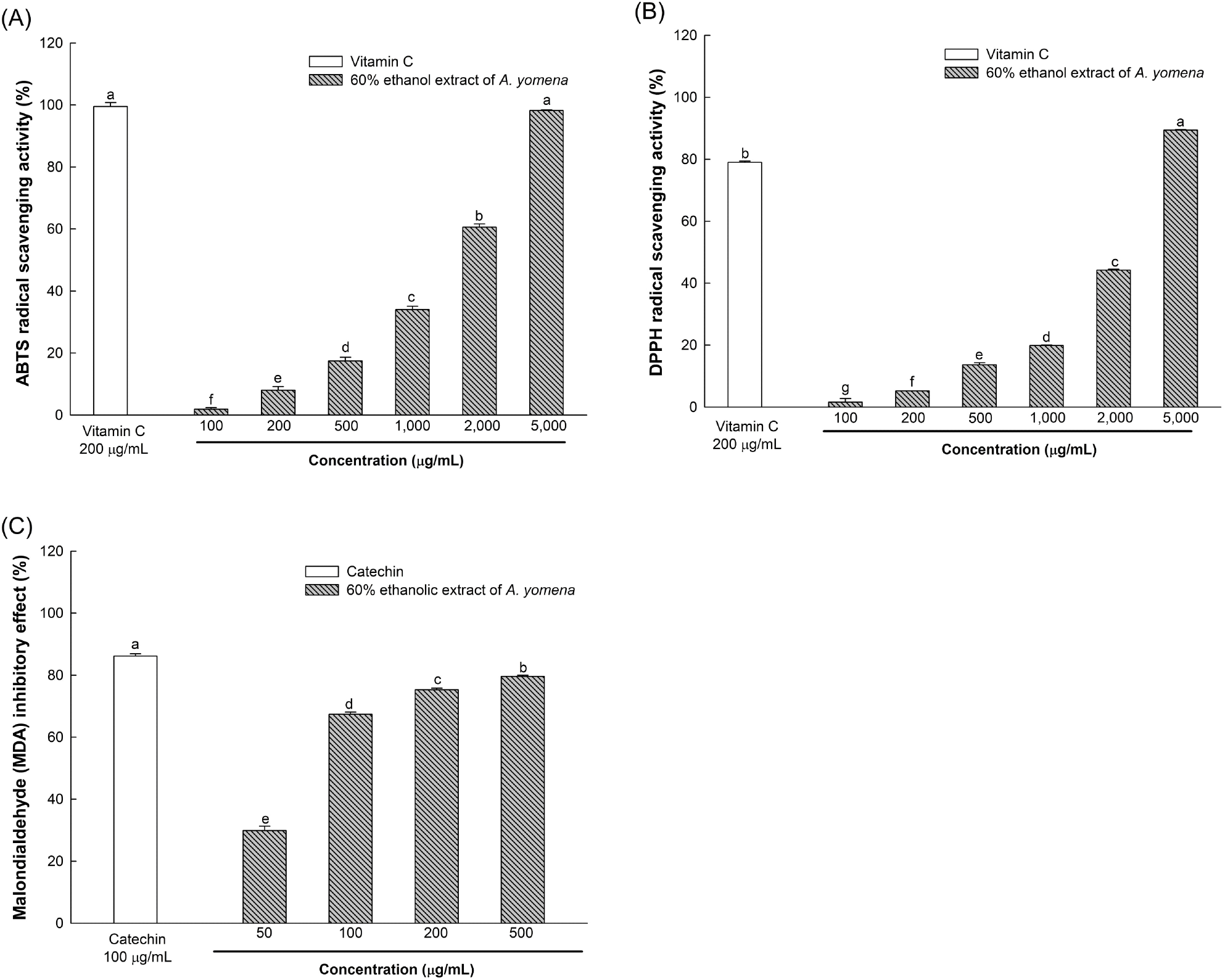
Polyphenols and flavonoids, being secondary metabolites of plants, contain hydroxyl and carboxyl groups within their molecules, enabling them to neutralize ROS such as •OH and H2O2, thereby averting ROS-induced reactions such as lipid peroxidation and protein oxidation (Zagoskina et al., 2023). Plants of the Asteraceae family, including A. yomena, harbor diverse phytochemicals such as lignan and saponin, which have been reported to enhance the endogenous antioxidative system and hinder free radical formation (Rolnik and Olas, 2021). Therefore, the superior in vitro antioxidative activity observed in the 60% ethanol extract of A. yomena is attributed to its elevated phenolic and flavonoid compound content compared to other ethanol extracts.
Brain tissues, rich in unsaturated fatty acids, are prone to substantial ROS generation via Fenton oxidation mediated by polyvalent metal ions. Excessive ROS production in the brain can lead to the accumulation of MDA, a lipid peroxide known to induce lesions through reactions with DNA bases or membrane degeneration, serving as an indicator of oxidative stress (Jeong et al., 2021). Conversely, phenolic compounds have been noted to inhibit ROS generation, such as •OH, thereby preventing MDA production in tissues (Han et al., 2020). Chlorogenic acid, a bioactive compound found in Asteraceae plants, is a hydroxycinnamic acid derivative formed between trans-cinnamic acids like caffeic acid and ferulic acid, and quinic acid (Rolnik and Olas, 2021). According to Park et al. (2021), A. yomena contains chlorogenic acid and ferulic acid, which have been shown to mitigate neurodegeneration by suppressing the expression of pro-inflammatory cytokines triggered by microglial cell activation. Therefore, the 60% ethanol extract of A. yomena, which inhibits MDA generation due to its phenolic compounds, is believed to shield nerve cells from oxidative stress.
H2O2, an endogenous ROS, oxidizes various macromolecules such as nucleic acids, lipids, and proteins. Its reaction with Fe2+, a reactive metal abundant in the brain, generates •OH, which is believed to be the primary agent of oxidative damage mechanisms. Consequently, H2O2 is widely employed to induce oxidative stress in cells (Ransy et al., 2020; Zhong et al., 2023). This study induced oxidative stress using H2O2 to assess the neuronal protective effect of the 60% ethanol extract of A. yomena against oxidative stress. Lee et al. (2017) found no cytotoxicity when 3T3-L1 cells were treated with 100-500 μg/mL of 70% ethanol extract of A. yomena, while Rhew et al. (2020) reported no cytotoxicity in RAW 264.7 cells treated with 125-500 μg/mL of 70% ethanol extract of A. yomena. Based on these findings, this study determined that concentrations of 10-200 μg/mL of the 60% ethanol extract of A. yomena were non-cytotoxic.
The viability of nerve cells against H2O2 was assessed using the MTT assay, which relies on the conversion of tetrazolium compounds to purple formazan by dehydrogenase generated in the mitochondria of living cells, as shown in Fig. 3(A) and (B) (Kuete et al., 2017).
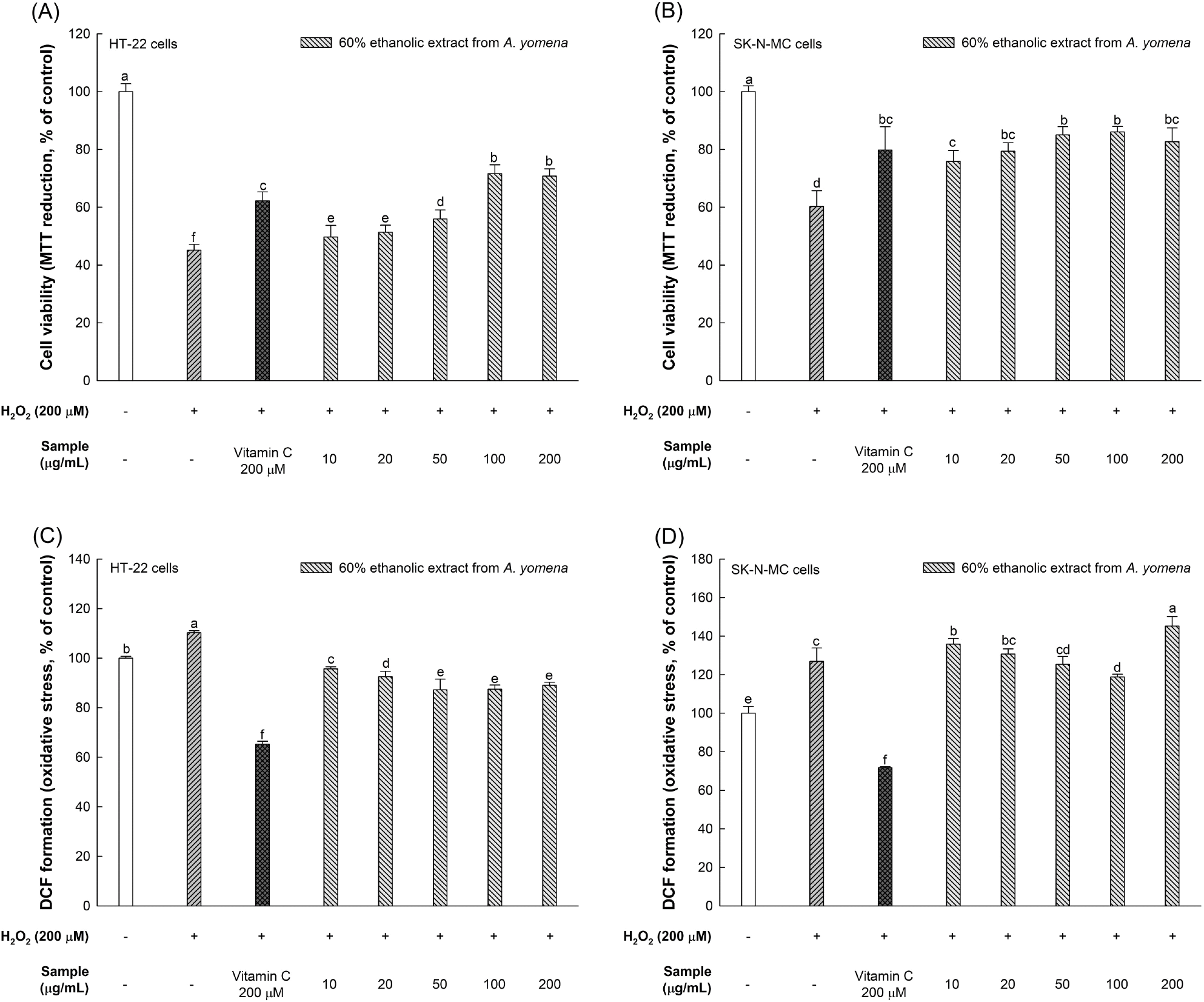
While the viabilities of HT-22 and SK-N-MC cells treated solely with H2O2 were 45.12% and 60.23%, respectively, those treated with 100 μg/mL of the 60% ethanol extract of A. yomena exhibited viabilities of 71.62% and 86.03%, respectively, demonstrating a significant increase in neuron viability.
The ROS content in HT-22 and SK-N-MC cells treated only with H2O2 was 110.26% and 126.86%, respectively. However, treatment with 100 μg/mL of the 60% ethanol extract of A. yomena reduced ROS content to 87.46% in HT-22 cells and 118.80% in SK-N-MC cells. However, the ROS content increased by about 25% when SK-N-MC cells were treated with 200 μg/mL of the 60% ethanol extract of A. yomena.
The brain’s susceptibility to ROS stems from its high concentration of unsaturated fatty acids, crucial for nerve cell signaling, coupled with a relatively low level of antioxidant enzymes compared to other tissues. Oxidative stress induced by ROS contributes to neurodegenerative diseases (ND) by harming neuronal nuclei and DNA (Han et al., 2020; Jeong et al., 2021). Conversely, polyphenol compounds mitigate oxidative stress-related ailments, including neurodegeneration, by intercepting electrons and hydrogen atoms from ROS to curb damage responses (Gentile et al., 2023). A. yomena is recognized for containing chlorogenic acid and rutin (Hwang et al., 2018; Park et al., 2021). Kim et al. (2020) observed diminished cell viability in SH-SY5Y neurons when treated with 300 μM H2O2, but the ethyl acetate fraction of A. yomena bolstered cell viability by reducing inflammatory factors such as cyclooxygenase-2 (COX-2) and interleukin-1β (IL-1β), and regulating the expression of apoptotic proteins like B-cell lymphoma 2 (Bcl-2), Bcl-2 associated X (BAX), and cysteine-aspartic proteases-9 (caspase-9). Seo (2020) noted that A. yomena extract augmented cell viability in C6 glioma cells due to its antioxidative properties. Hence, drawing from the findings of this study and previous research, it is inferred that the phytochemicals present in A. yomena function as antioxidants, mitigating oxidative stress and conferring neuroprotective benefits. Consequently, A. yomena holds promise as a preventive measure against ND.
HLPC analysis of the 60% ethanol extract of A. yomena, which exhibited antioxidant and neuronal protective effects, was conducted. The peaks appearing at retention times (RT) of 13.933 min and 16.400 min at 254 nm were identified as the main bioactive substances (Fig. 4(A)). By comparing RT and UV-Vis spectra with standard materials using the same method as the sample, it was confirmed that the two peaks corresponded to chlorogenic acid (similarity: 999.98) and rutin (similarity: 999.16), respectively. Quantitative analysis revealed chlorogenic acid and rutin contents in the sample of 20.74 μg/mg and 3.60 μg/mg of dried weight, respectively (Fig. 4(B) and (C)).
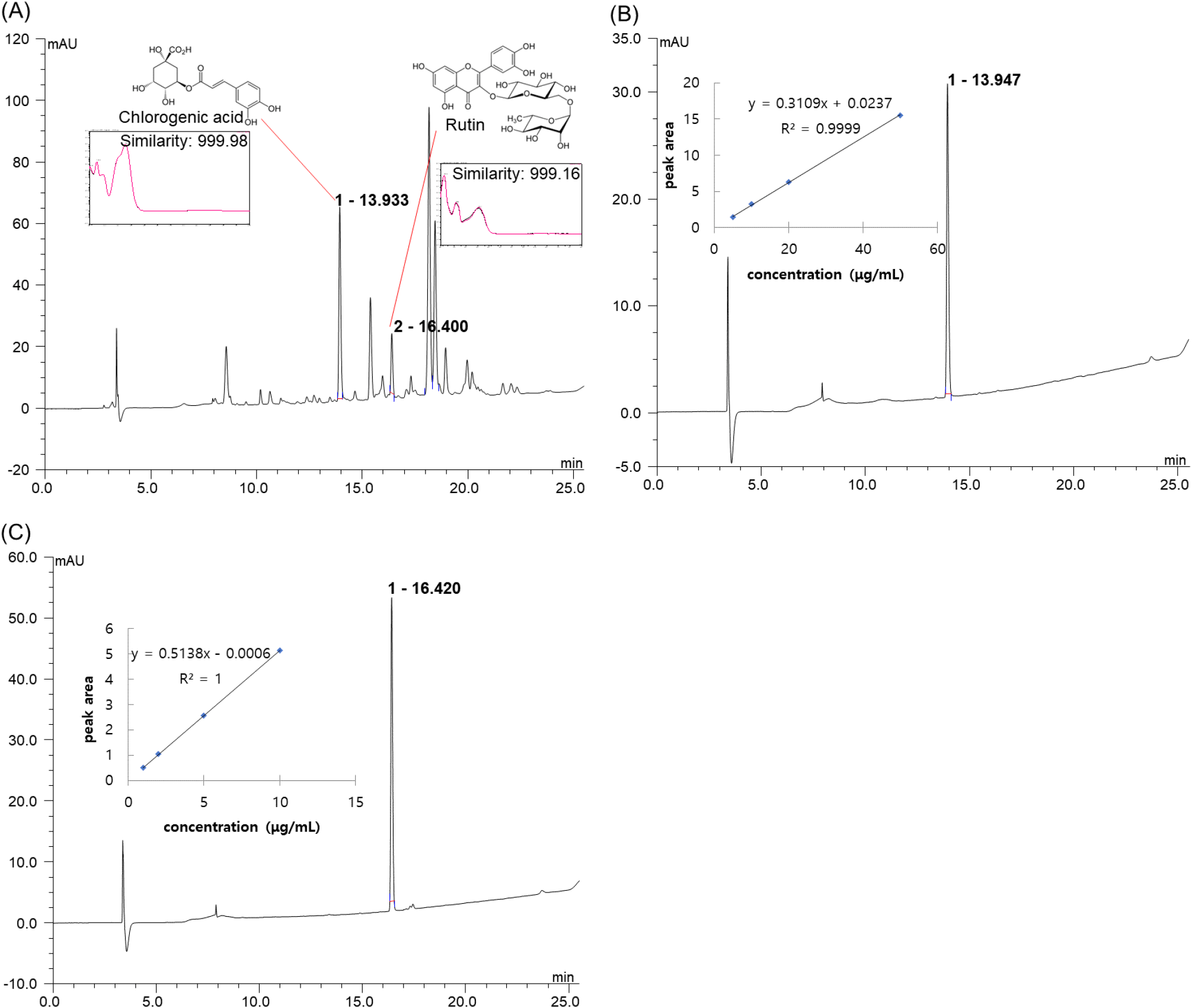
The brain’s vulnerability to ROS, which leads to mitochondrial damage, reduces intercellular ATP levels, resulting in cell dysfunction and death, thereby inducing ND (Heitman and Ingram, 2017). Chlorogenic acid, a polyphenol compound produced via the shikimic acid pathway during plant aerobic respiration, scavenges radicals directly owing to its polyhydroxyl structure. It activates the nuclear factor erythroid 2-related factor 2 (Nrf2) pathway, thereby reducing MDA levels by upregulating the expression of endogenous antioxidants such as superoxide dismutase, catalase (CAT), and glutathione peroxidase (GSH-Px) (Wang et al., 2022). Moreover, chlorogenic acid exerts a neuroprotective effect by penetrating the blood-brain barrier and acting as a direct antioxidant in the brain. It mitigates oxidative stress induced by H2O2 in PC12 cells and attenuates Aβ-induced cell death in SH-SY5Y cells (Heitman and Ingram, 2017).
Flavonoids are known to enhance cognitive function by protecting neurons, promoting neuronal differentiation, and reinforcing synaptic plasticity. Rutin, a flavonol glycoside comprising rutinose and quercetin, possesses pharmacological activity and serves as a potent antioxidant with anti-inflammatory, antidepressant, and neuroprotective effects (Ramalingayya et al., 2017). Rutin suppresses the activation of inflammatory cytokines and microglial cells associated with neuroinflammation and plays a neuroprotective role in rats with CA3 region neuronal damage induced by trimethyltin, which causes spatial memory impairment (Sivanantham et al., 2018). Furthermore, rutin protects BMG-1 cells from oxidative stress and alleviates SH-SY5Y cell death induced by tau (Kumar et al., 2014; Sun et al., 2021). Therefore, the antioxidative activity and neuronal protective effects observed in the 60% ethanol extract of A. yomena in this study are attributed to major phenolic compounds, such as chlorogenic acid and rutin, present in A. yomena.
4. Conclusions
This study aimed to investigate the antioxidative activity and neuronal protection effects of A. yomena while identifying major bioactive substances. The 60% ethanol extract of A. yomena demonstrated notably robust antioxidant activity. Moreover, it exhibited a significant cytoprotective effect on HT-22 (mouse hippocampal neuronal cell line) and SK-N-MC (human neuroblastoma cell line) cells subjected to oxidative stress induced by H2O2, leading to enhanced cell survival and suppression of oxidative stress. HPLC analysis revealed that chlorogenic acid and rutin were the primary bioactive substances in the 60% ethanol extract of A. yomena. Based on these findings, the in vitro antioxidative activity and neuronal protection effects observed in the 60% ethanol extract of A. yomena are attributed to major polyphenolic compounds, including chlorogenic acid and rutin. Future research will delve into exploring the potential of A. yomena extracts as an industrial material for preventing ND through in vivo and ex vivo studies using animal models.