1. Introduction
Paprika (Capsicum annuum L.) is a widely consumed vegetable known for its vibrant colors, distinct flavor, and nutritional benefits. It is a perishable fruit, and maintaining its quality and safety during post-harvest storage and distribution is challenging. Among the various factors influencing paprika quality, storage temperature plays key role (Rao et al., 2011). Most of the physical, biochemical, microbiological, and physiological reactions that contribute to the deterioration of fruit quality depend greatly on storage temperature (Lama et al., 2020). While storage at low temperatures extends the shelf-life, prolonged exposure to low temperatures causes chilling injuries (CI), such as pitting, development of sunken areas on the fruit, and increased susceptibility to rotting and decay (Afolabi et al., 2023; O’Donoghue et al., 2018). In contrast, high storage temperatures above 10°C accelerate fruit senescence, potentially leading to increased decay and reduced marketability of paprika (Lama et al., 2020; Xu et al., 2023). Therefore, determination of the optimal storage temperature is necessary to minimize CI and maintain the quality attributes of paprika.
Prolonged exposure to suboptimal temperatures leads to the accumulation of reactive oxygen species (ROS). ROS, including superoxide radicals, hydrogen peroxide, and hydroxyl radicals, induce oxidative stress, leading to mitochondrial dysfunction, enzyme inactivation, and lipid peroxidation (Tian et al., 2013). CI and various postharvest disorders in fruits and vegetables are often associated with oxidative damage (Meitha et al., 2020). Furthermore, fruit ripening and senescence are closely associated with production of ROS (Jimenez et al., 2002; Rosalie et al., 2018; Tian et al., 2013). Plants use non-enzymatic and enzymatic antioxidant systems, including ascorbate peroxidase, superoxide dismutase, catalase (CAT), and peroxidase, to detoxify ROS (Hodges et al., 2004; Meitha et al., 2020; Tian et al., 2013). However, high ROS levels accelerate fruit ripening, senescence, and associated conditions, including CIs and decay (Biswas et al., 2016; Jimenez et al., 2002; Qin et al., 2009; Stadtman, 1992; Tian et al., 2013). Considering the involvement of ROS in ripening, senescence, and chilling stress, ROS detoxification by antioxidant enzymes may be correlated with fruit storability. The effect of different storage temperatures on antioxidant activity was studied in various produce, such as peaches, mangoes, and various berries (Juan et al., 2011; Piljac-Žegarac and Šamec, 2011; Rosalie et al., 2018; Šamec and Piljac-Žegarac, 2011). Additionally, the impact of induced antioxidant activity on ripening attributes and shelf-life in various produce, including sweet peppers, pears, and cucumbers, has been investigated (Li et al., 2020; Lin et al., 2022; Rao et al., 2011). However, the collective effect of storage temperature on the antioxidant system and its association with storability, particularly in paprika, remains unknown.
In this study, we assessed the storage attributes, antioxidant enzyme activity, and gene expression levels in paprika at various storage temperatures to determine the ideal storage conditions that influence its antioxidant activity, thereby affecting its storage and distribution.
2. Materials and methods
Paprika fruits (Capsicum annuum L. cv Sirocco, red color) were harvested at approximately 80-85% maturity, with each fruit weighing about 200 g, from hydroponically cultivated plants in Gimje, Jeollabuk-do, Korea, in April 2023. The fruits were initially stored in covered cardboard boxes at 4, 10, and 20°C for 14 d and then at 20°C for an additional 5 d (14+5 d; retail conditions). Relative humidity was maintained at 90±5% during the storage period. Fifteen boxes, each containing 24 fruits, were used in the study.
Briefly, 24 fruits were sampled per storage temperature to determine the fruit storage attributes. The fruits were weighed to determine weight loss using an electronic weighing balance. Firmness was analyzed using a texture analyzer (TA Plus Lloyd Instruments Ltd., Fareham, Hamshire, UK) equipped with a 5-mm plunger head (diameter) at a speed of 2 mm/s. Total soluble solid content (SSC) was analyzed using a digital refractometer (PAL-1, Atago Co. Ltd., Tokyo, Japan). Fruit pitting, decay, and marketability were expressed as the percentages of fruits that exhibited pitting, decay, and marketability, respectively. Stalk browning and shriveling were expressed as indices: 0 = no stalk browning/no shriveling, 1 = fruits with <50% stalk browning/shriveling, and 2 = fruits with >50% stalk browning/shriveling. Then, fruit marketability was analyzed using a panel of two people. The final reported storage attributes were obtained from three independent replicates per treatment per day.
Sample extraction was performed following the procedure described by Vasco et al. (2018) with slight modifications. Briefly, freeze-dried fresh fruit samples were ground, and the resulting powder (500 mg) was extracted twice at 25±2°C for 2 h under constant shaking, first with a 20 mL mixture of methanol and water (50:50, v/v) and then with a 20 mL mixture of acetone and water (70:30, v/v). Thereafter, the extracts were centrifuged at 4,000 rpm for 15 min to remove the supernatant, and the filtered extracts were used to assess the total phenolic and antioxidant activities. All reagents were purchased from Sigma–Aldrich (St. Louis, MO, USA). Total polyphenol content was measured using a modified version of the method described by Singleton and Rossi (1965). Briefly, 100 μL of extract or standard was reacted with 100 μL of 1 N Folin-Ciocalteu reagent for 3 min, followed by the addition of 1 mL of 2% sodium carbonate solution. The mixture was incubated for 30 min and the absorbance was read at 726 nm using a microplate reader (EPOCH2, BioTek Instruments Inc., Winooski, VT, USA). The results are expressed as gallic acid equivalents (GAE, mg/g DW).
1,1-Diphenyl-2-picrylhydrazyl (DPPH) radical scavenging activity of the samples was assessed using the method described by Dietz et al. (2005). Briefly, 20 μL of extract was mixed with 180 μL of 0.18 mM DPPH, and then vortexed. After standing for 20 min, the absorbance was measured at 515 nm using a microplate reader. Methanol without DPPH radicals was used as the blank reference, and the absorbance was converted to DPPH radical scavenging activity. Additionally, 2,2-azino-bis-3-ethylbenzothiazoline-6-sulfonic acid (ABTS) radical-scavenging activity was evaluated according to the method developed by Re et al. (1999), with some modifications. Briefly, an ABTS stock solution (7 mM) was prepared from 7 mM ABTS and 2.45 mM potassium persulfate solution and kept in the dark for 16 h. The reagent was adjusted with ethanol until the absorbance at 734 nm reached 0.7±0.02 at ambient temperature (25±2°C). Thereafter, 20 μL of each extract was mixed with 180 μL of the ABTS+ solution, and the absorbance at 734 nm was measured after 10 min at ambient temperature (25 ±2°C). The DPPH and ABTS radical scavenging activity were expressed as Trolox equivalent (TE) antioxidant capacity (μmol TE /g dw).
Total RNA was extracted from freeze-dried fresh fruit samples using the RNeasy Plant Mini kit (Qiagen, Valencia, CA, USA). Subsequently, 1 μg of RNA was used to synthesize cDNA with a QuantiTect Reverse Transcription kit (Qiagen). The resulting cDNA was utilized for Quantitative real-time PCR (qRT-PCR) as described by Park et al. (2021). Target genes were amplified on a CFX96 TouchTM Real-Time PCR Detection System (Bio-Rad, Hercules, CA, USA) using the iQTM SYBR Green Supermix (Bio-Rad) with specific primers (Table 1). The qRT-PCR conditions were as follows: 95°C for 30 s, followed by 40 cycles of 95°C for 10 s and 55°C or 58°C for 40 s. The relative gene expression was calculated using the 2−ΔΔCT method and normalized to that of the housekeeping genes actin and elongation factor 1. The qRT-PCR was performed using at least three biological replicates and two technical replicates.
Data are presented as the mean±standard error. Samples were subjected to analysis of variance, and significant differences were determined using Duncan’s multiple range test. Significant differences in fruit pitting were determined using analysis of variance (ANOVA), followed by t-test for comparisons between groups. All analyses were performed using SAS v.9.2 (SAS Institute, Cary, NC, USA).
3. Results and discussion
Fruits stored at 10°C had lower pitting rates than those stored at 4°C on days 14 and 14+5 (Fig. 1(A), (B)). On day 14+5, while more than 85% of the fruits showed pitting at 4°C, it was only observed in 25% of the fruits stored at 10°C (Fig. 1(B)). Notably, storage at 20°C did not cause any pitting (data not shown). Fruits stored at 20°C exhibited stalk browning symptoms on the day 7 of storage, whereas these symptoms were delayed for 7 d in fruits stored at 4 and 10°C (Fig. 1(C)). Stalk browning increased in all treatment groups under retailer conditions. However, fruits at 10°C had the lowest, whereas those at 20°C had the highest stalk browning index. Fruits at 4°C showed intermediate levels of stalk browning index (Fig. 1(C)). Shriveling symptoms were significantly lower in fruits stored at 4 and 10°C than in those stored at 20°C (Fig. 1(D)). However, no difference in shriveling symptoms was observed between fruits stored at 4 and 10°C (Fig. 1(D)). Fruits stored at 20°C tended to decay by day 14, whereas those stored at 4 and 10°C did not exhibit any decay symptoms until day 5 under retail conditions (Fig. 1(E)). Notably, fruits stored at 10°C exhibited the lowest decay percentage compared to those stored at 4 and 20°C (Fig. 1(E)). These findings are consistent with those of previous studies that highlighted development of CI symptoms in paprika stored at temperatures lower than 7°C (Lama et al., 2020; O’Donoghue et al., 2018; Park et al., 2023; Rao et al., 2011). Furthermore, there was largely no significant difference in weight loss, firmness, and SSC observed between the fruits stored at 4 and 10°C (Table S1). However, fruits stored at 20°C were less firm, with higher SSC and weight loss compared to those stored at 4 and 10°C (Table S1). Fruit marketability decreased during the storage period in all treatment groups. Notably, fruits stored at 20°C exhibited the lowest marketability during storage (Fig. 1(F)). No difference in fruit marketability was observed between 4 and 10°C until day 14 of storage; however, fruits stored at 10°C had significantly higher marketability than those stored at 4°C under retail conditions (Fig. 1(F)). These results suggest that storing paprika at 10°C proved to be the most effective condition for preserving its quality (Fig. 1).
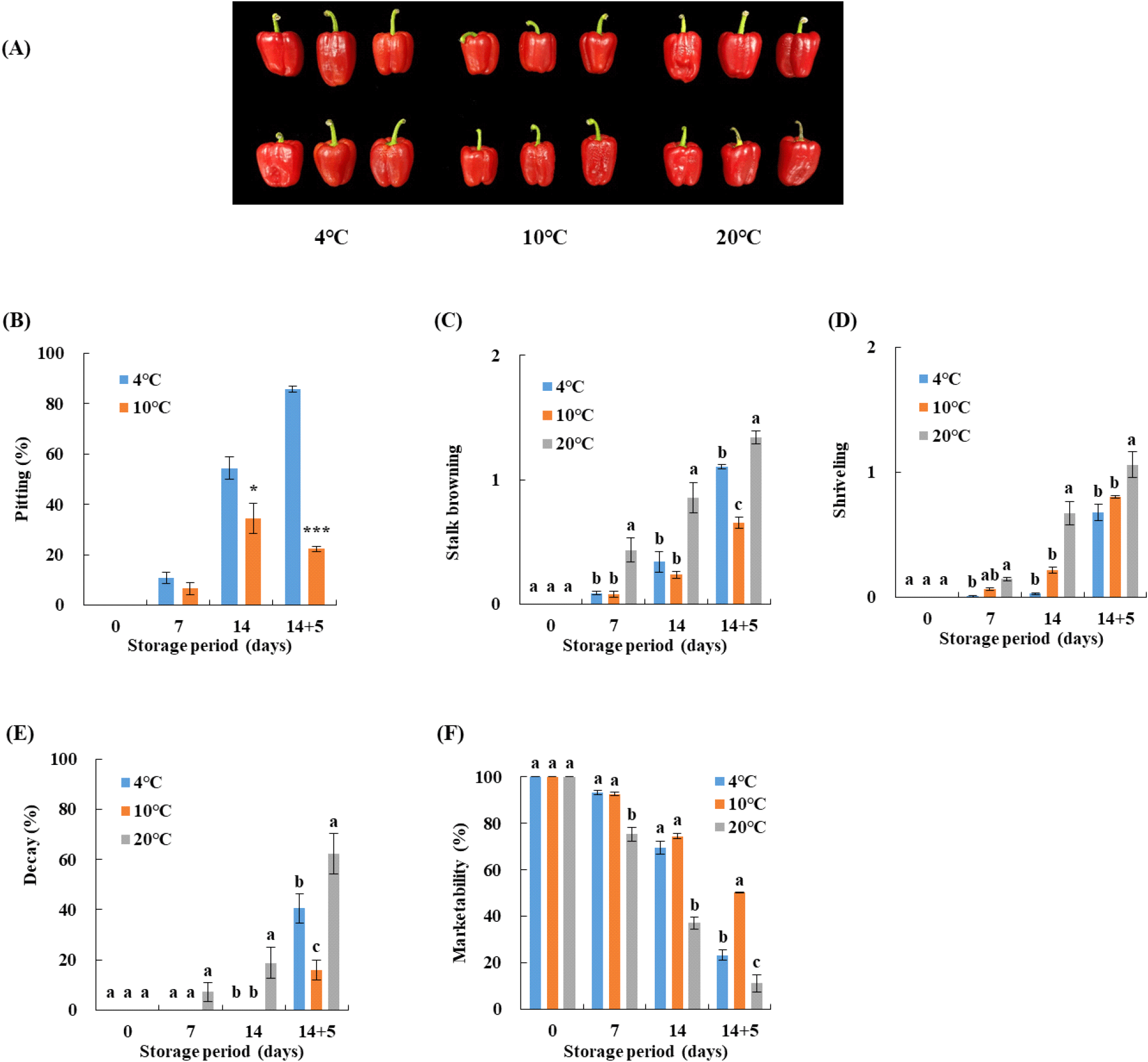
Fruits stored at 10°C exhibited higher ABTS activity than those stored at 4 and 20°C on days 14 and 14+5. Additionally, ABTS activity in fruits stored at 4°C was consistently lower than or equal to that in fruits stored at 20°C, depending on the specific storage day (Fig. 2). In contrast, DPPH activity did not significantly differ between the treatment groups, except on day 7 when fruits stored at 20°C showed lower DPPH activity than those stored at 4 and 10°C (Fig. 2). Additionally, the effect of storage temperature on total phenolic content was significant on day 14+5, with fruits stored at 10°C exhibiting the highest total phenolic content, whereas those stored at 4°C exhibiting the lowest phenolic content. The total phenolic content of fruits stored at 20°C fell between the levels observed at 4 and 20°C (Fig. 2). Furthermore, on day 7, fruits stored at 10°C exhibited higher expression levels of genes encoding catalase (catalase 2) and peroxidase (peroxidase 51) than those stored at 4 and 20°C. Although the expression levels of catalase were higher in fruits stored at 4°C than in those stored at 20°C, the expression levels of peroxidase were not significantly different between the treatment groups on day 7 (Fig. 3). Moreover, the expression levels of these genes tended to decrease after day 7, regardless of the storage temperature (Fig. 3). These results suggest that storage temperature plays a significant role in modulating the antioxidant capacity in paprika, similar to various other fruits, such as peach, mango, and various berries (Juan et al., 2011; Piljac-Zegarac and Samec, 2011; Rosalie et al., 2018, Samec and Piljac-Zegarac, 2011).
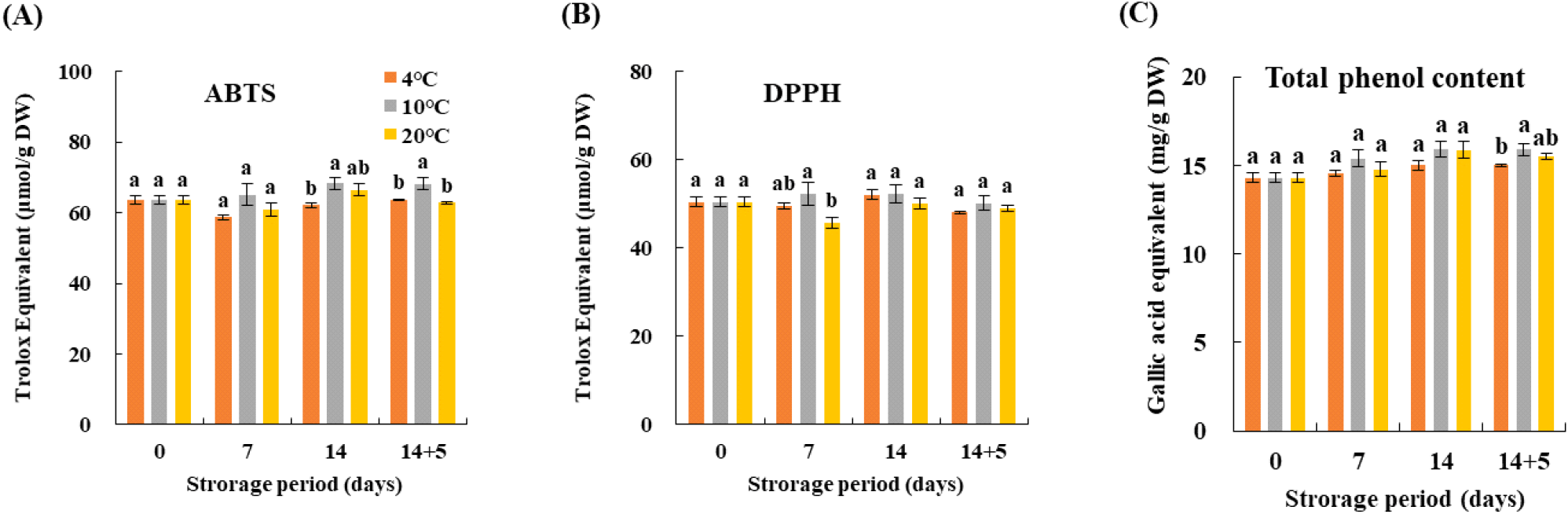
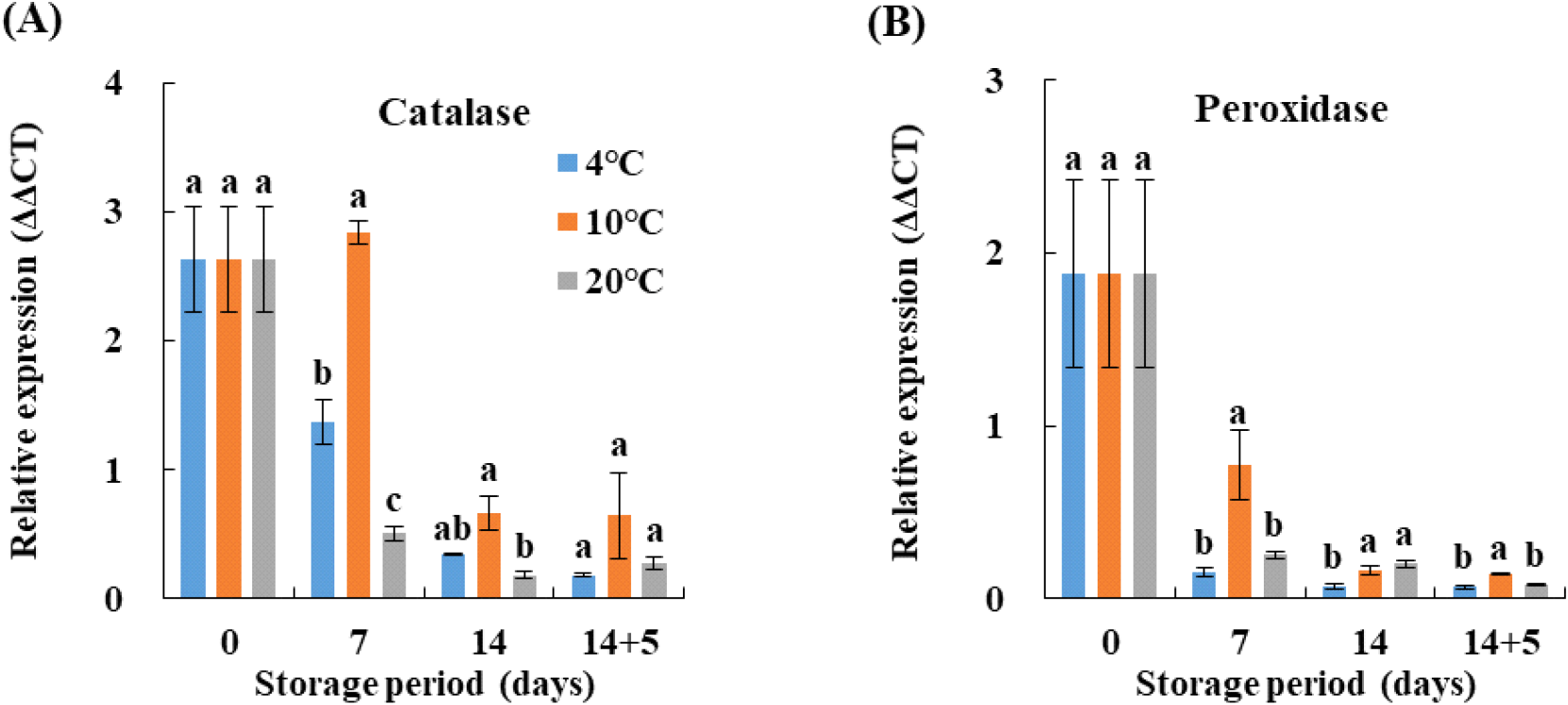
Enhanced ROS scavenging, achieved via increased antioxidant enzyme activity, is associated with delayed ripening and prolonged shelf-life in different produce. For example, sweet peppers treated with salicylic acid and calcium chloride exhibit delayed ripening and extended shelf-life due to enhanced antioxidant enzyme activity (Rao et al., 2011). A similar effect was observed in pears treated with acibenzolar-S-methyl, in which the increased antioxidant enzyme activity effectively delays the senescence process (Li et al., 2020). Furthermore, induction of antioxidant activity improves the fruit resistance to senescence. Lin et al. (2022) reported that the regulation of ROS balance and improvement in antioxidant capacity alleviate CI in cucumbers. Similarly, increased antioxidant enzyme activity reduces CI and decay in sweet peppers (Hanaei et al., 2022). Moreover, fruit quality attributes were found to significantly correlate with antioxidant enzyme activities (Sang et al., 2022). In this study, the enhanced antioxidant capacity observed at 10°C likely contributed to the reduced pitting, stalk browning, shriveling, and decay and improved marketability of paprika.
4. Conclusions
This study revealed the crucial roles of storage temperature in preserving the quality and enhancing the antioxidant capacity of paprika. Our findings revealed storage at 10°C as an effective strategy to extend the shelf-life, minimize the quality deterioration, and enhance the antioxidant properties of paprika, ultimately improving the post-harvest handling and marketing of this valuable agricultural product. However, further studies are necessary to understand the complex association between storage temperature and the antioxidant system in paprika. Understanding the molecular mechanisms underlying the storage temperatures and antioxidant system could provide valuable insights for developing targeted strategies to optimize paprika storage conditions.