1. Introduction
Salmonella is one of the most common and serious foodborne pathogens worldwide owing to its ubiquitous existence and excellent survival in agricultural environments (Oh and Park, 2017; Scallan et al., 2011). According to the Centers for Disease Control and Prevention (CDC), Salmonella causes approximately 1.35 million outbreaks and 26,500 hospitalizations annually in the USA (CDC, 2021). These frequent Salmonella outbreaks are associated with its persistent survival under environmental stress conditions in food processing facilities due to its biofilm formation on the food contact and/or food surfaces (Park et al., 2013; Reij et al., 2004; Rodrigues et al., 2011).
A biofilm is defined as “an aggregation of microorganisms embedded within a matrix of extracellular polymeric substance (EPS)” (Vert et al., 2012). Once Salmonella contaminated and reversibly attached on the food contact surface, such as polypropylene (PP), rubber, and glass, it mediates an irreversible attachment by producing fimbriae (cellulose and curli) or flagella. Subsequently, the attached Salmonella forms bacterial aggregates and synthesizes EPS to develop the complex structure of a mature biofilm (Suslow et al., 2000). Salmonella biofilm formed on the industrial food facilities can lead to numerous Salmonella outbreaks due to its cross-contamination during food processing (Corcoran et al., 2014). Moreover, Salmonella embedded within its biofilm can survive during harvest, transport, processing, and storage because it plays an essential role in protecting the bacteria and reducing the efficacy of sanitizers used for washing (e.g., chlorine, hydrogen peroxide, vinegar, and iodine) (Bassam et al., 2005; Vanessa et al., 2012).
The formation and maturation of Salmonella biofilm are influenced by several environmental factors such as surface topology, temperature, pH, and nutrients (Zhao et al., 2017). Salmonella can form its biofilms on various food contact surfaces such as polyvinyl-chloride, polyethylene, polystyrene, glass, rubber, and PP (Garcia et al., 2017; Islam et al., 2019; Tiwari et al., 2013). In particular, PP is one of the most popular food packaging materials that has been approved by the Food and Drug Administration (FDA) (Wu et al., 2021). Thus, there is an increasing interest in searching for an effective strategy for controlling Salmonella biofilm on PP surface.
Bacteriophages (phages) have emerged as promising biocontrol alternatives for controlling foodborne pathogen and their biofilm due to their ubiquitous existence, specificity, and bactericidal effect (Azeredo et al., 2021; Choi et al., 2017; Endersen et al., 2014; Sarhan and Azzazy, 2015). Phages can specifically infect desired target pathogens using the lytic cycle. Since 2006, several commercial phages have been approved by the FDA as “food additives”; e.g., SalmoFreshTM (Intralytix Inc.), PhageGuard STM (Micreos Co., Wageningen, Netherlands), SalmoPro® (Phagelux Inc., Shanghai, China), and SalmoFREE® (LANXESS Inc, Cologne, Germany). Additionally, some phages have recently been considered as potential biocontrol candidates to control biofilm due to their lytic activity and EPS degrading ability via depolymerase activity (Fu et al., 2010; Meng et al., 2011). Despite numerous studies on phages, only few Salmonella phages have been reported and employed for controlling Salmonella biofilm (Iibuchi et al., 2010; Tiwari et al., 2013) on food contact surfaces, such as polystyrene, glass, rubber, and stainless steel. Thus, the purpose of this study was to isolate, purify, and characterize a lytic S. Typhimurium-specific phage as an effective biocontrol agent for S. Typhimurium biofilms.
2. Materials and methods
Salmonella Typhimurium ATCC 13311 was obtained from the American Type of Culture Collection (ATCC) and was cultivated using tryptic soy broth (TSB, Difco Laboratories Inc., Sparks, MD, USA) by incubating at 37°C for 16 h. After centrifugation of the bacterial suspension at 7,000 ×g for 4 min, the bacterial pellet was washed with phosphate-buffered saline (PBS 1×, pH 7.4, Life Technologies Co., Carlsbad, CA, USA) by repeating three times. The concentration of the bacterial suspension was adjusted to 8 log CFU/mL based on its optical density (OD) at 600 nm.
For phage isolation, the mixture of bacterial suspension (1%, v/v), TSB (250 mL), and the sample acquired from water used in a slaughterhouse (25 mL, Gumi, Gyeongsangbuk-do, Korea) was incubated at 37°C for 16 h. After centrifugation at 2,400 ×g for 20 min and filtration using a 0.22 μm pore size-filter (Advantec Toyo Kaisha Ltd., Tokyo, Japan), the presence of the phage in the filtrate was confirmed using plaque assay following this procedure; TA soft agar (0.4% agar) containing 100 μL of the phage suspension and 200 μL of the bacterial suspension (∼8 log CFU/mL) was poured onto pre-solidified tryptic soy agar (TSA) plates and incubated at 37°C for 16 h (plaque assay). Once the plaques of ST phage were visible on the TSA plates, they were eluted with 1 mL of sodium chloride-magnesium sulfate (SM) buffer (50 mM/L Tris-HCl, 100 mM NaCl, and 10 mM MgSO4, pH 7.5).
The isolated phage acquired from the plaques was propagated by two-step incubation procedures (Gwak et al., 2018; Kim et al., 2022). First, 1% (v/v) of S. Typhimurium ATCC 13311 suspension and TA broth (8 g/L nutrient broth, 5 g/L NaCl, 0.2 g/L MgSO4, 0.05 g/L MnSO4, and 0.15 g/L CaCl2) was incubated at 37°C for 2 h, and then the eluted phage solution was added followed by incubation at 37°C for 2 h. After centrifugation of the mixture at 6,000 ×g for 20 min and filtration using a 0.22 μm filter, the filtered supernatant was used instead of phage solution. This propagation was rerun by increasing volumes of host bacteria and TA broth by 3, 20, and 200 mL. Afterward, the phage was purified by polyethylene glycol 6000 (Sigma-Aldrich Co., St. Louis, MO, USA) precipitation and ultracentrifugation at 7,200 ×g for 120 min based on the previously described method (Lee et al., 2020). Finally, the obtained opalescent band was dialyzed by incubation into 2 L of SM buffer for 4 h (Kim et al., 2021). The final titer of the purified S. Typhimurium-specific phage (hereafter referred to as ST phage) was determined using plaque assay.
The morphological characteristic of ST phage was investigated by observing using transmission electron microscopy (TEM, H-7700, Hitachi Ltd., Tokyo, Japan) (Kim et al., 2021). ST phage was deposit on a copper grid by dipping into ST phage suspension for 1 min. Once ST phage was treated with 1% uranyl acetate (Sigma-Aldrich Co.), TEM analysis was conducted at a voltage of 100 kV with 10,000× magnification.
To evaluate the bactericidal activity of ST phage, S. Typhimurium suspension (∼7 log CFU/mL) was treated with ST phage at multiplicities of infection (MOIs) of 0.01, 1, and 100, and then incubated at 37°C for 10 h. SM buffer was used in the control for comparison. The viable bacterial concentration was enumerated using xylose lysine deoxycholate (XLD, Difco Laboratories Inc.) plate count method at every 2-h interval.
One milliliter of overnight culture of S. Typhimurium (∼6 log CFU/mL) was loaded into a sterilized polypropylene (PP) tube. It was incubated at 37°C for 2 days under static condition to form biofilm on PP. TSB without bacterial suspension was used as a negative control. Next, the PP tube was washed thrice with filtered distilled water (FDW) to eliminate unattached S. Typhimurium. After drying the washed tube for 1 h, biofilm formation on the PP tube was confirmed via optical microscopy (Singh et al., 2016). Subsequently, the PP tube containing S. Typhimurium biofilm was used for phage treatment (indicated in 2.5.2. section).
Each PP tube was submerged into 5 mL of ST phage solution with an MOI of 100 and incubated for 2 h with gentle agitation. PBS was used instead of phage treatment as a negative control. Phage treated tube was washed thrice with FDW. S. Typhimurium biofilm on PP surface was stained with 2 mL of crystal violet (CV, Sigma-Aldrich Co.) followed by incubation at 22°C for 15 min. The CV complex incorporated into S. Typhimurium biofilm was dissolved by adding 1 mL of ethanol:acetone (8:2, v/v) solution before measuring the OD at 600 nm using a spectrophotometer (Agilent Technologies Inc., Santa Clara, CA, USA). Further, 5 mL of PBS was added to the other tube containing S. Typhimurium biofilm and the tube was vortexed vigorously. The detached S. Typhimurium from the biofilm was enumerated using XLD (Difco Laboratories Co.) agar plate count method at 30 min intervals to determine the biofilm population.
Experiments were conducted in triplicate, and data were expressed as the mean±standard deviation. Statistical analysis was performed using InStat V.9 (GraphPad, San Diego, CA, USA). One-way analysis of variance was applied for the comparison of data among groups. Differences among the mean values were analyzed by the Ducan’s multiple range test, and p values of ⟨0.05 were considered to indicate statistical significance.
3. Results and discussion
ST phage was isolated from rinsing water obtained from rinsing water obtained from a slaughterhouse. It formed a clear round plaque with a diameter of 8.1±0.7 mm after incubation (Fig. 1(A)). After phage isolation, its initial concentration was 4.2±0.2×104 plaque-forming unit (PFU)/mL (data not shown). After high-titer propagation, ST phage was increased to the concentration of ∼5 (Fig. 1(B)), ∼6 (Fig. 1(C)), and ∼8 log PFU/mL (Fig. 1(D)) as the amount of medium and S. Typhimurium increased to 3 mL, 20 mL, and 200 mL. ST phage was finally propagated and purified at a high concentration of (3.5±0.4)×1011 PFU/mL. TSA and XLD agar plates inoculated with the purified ST phage did not exhibit any bacterial colonies (Fig. S1). The guidelines of the United States Department of Agriculture (USDA) and FDA (21CFR 172.785) to use phages as food additives included criteria regarding the high microbial purity and production titer (>10 log PFU/mL) of the isolated lytic phages (Lang, 2006; Oliveira et al., 2012). As as our propagated and purified phage met these requirements, ST phage is suitable for use as a biocontrol agent in food products.
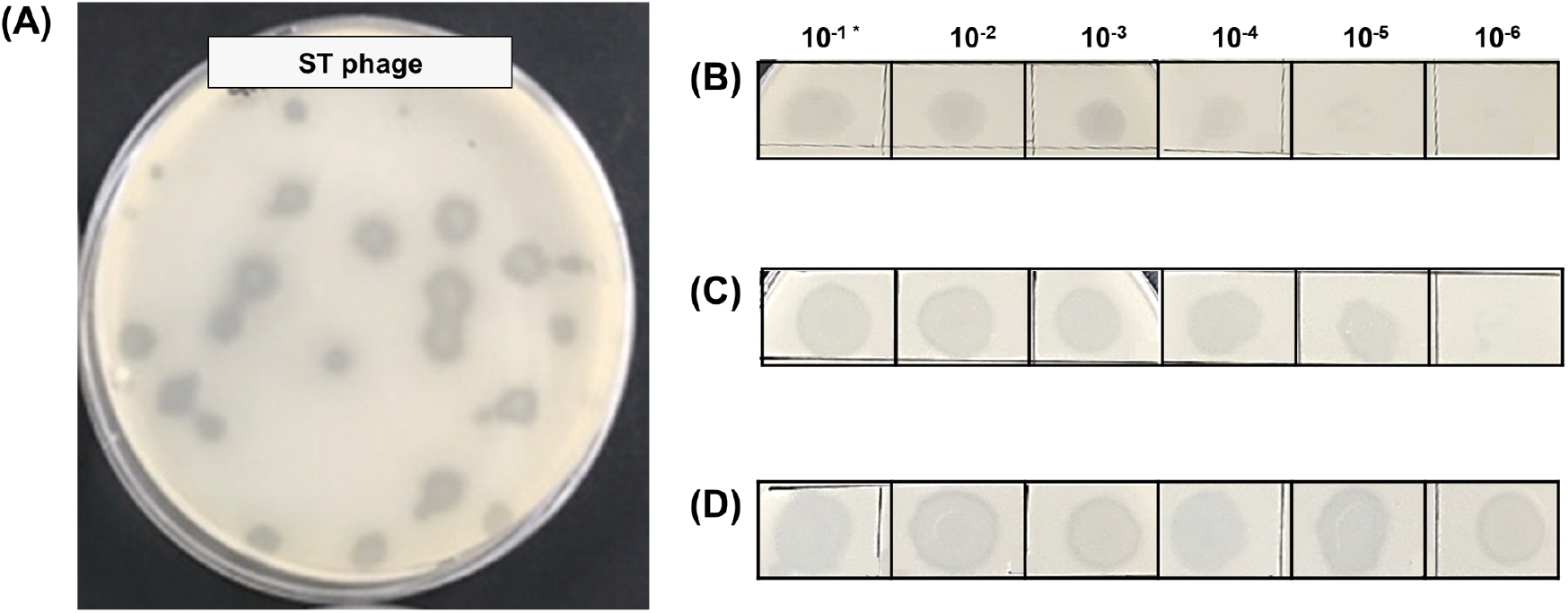
TEM analysis revealed that ST phage had an icosahedral head with a length of 101.1±5.7 nm and contractile tail with a length ranging from 80.2 nm to 125.5 nm (Fig. 2). Tailed phages are generally divided into several families based on their tail morphology (Zinke et al., 2022). According to the International Committee on Taxonomy of Viruses, Podoviridae and Autographiviridae have a short tail, whereas Siphoviridae, Drexlerviridae, and Demerecviridae have a flexible, long, and non-contractile tail. Unlike these phages, our ST phage was categorized as a myophage, which include families, such as Myoviridae, Ackermannviridae, Chaseviridae, and Herelleviridae, due to its possession of an icosahedral head and long, contractile tail (Zinke et al., 2022). The head and tail lengths of ST phage were longer than those of other Salmonella myophages, such as pSal-SNUABM-02 (Kwon et al., 2020), LPST94 (Islam et al., 2020), PS5 (Duc et al., 2020), and ZCSE2 (Mohamed et al., 2020). In contrast, the tail length of ST phage was shorter tail length than those of phage SPHG3 (Kalatzis et al., 2016) and LSE7621 (Liu et al., 2020). Thus, ST phage was proposed to be a newly isolated Salmonella phage based on its distinct morphological differences when compared to other Salmonella myophages.
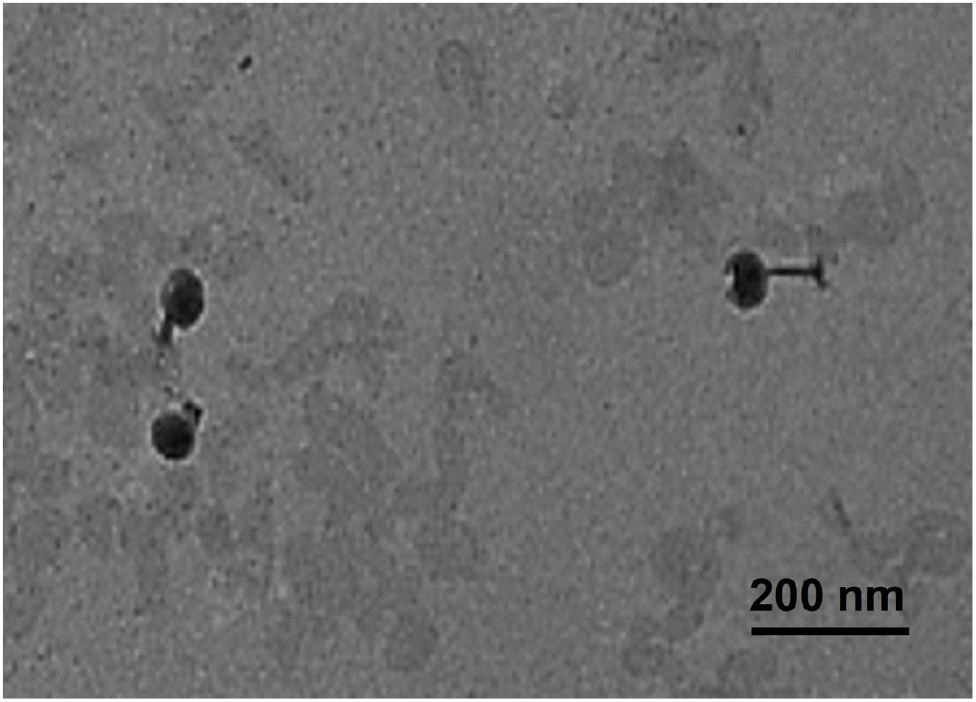
To determine the ideal conditions before its application on PP surface, the bactericidal effects of ST phage were compared at various MOIs of 0.01, 1.0, and 100 (Fig. 3). After 2 h of ST phage treatment, a significant decrease in S. Typhimurium population was initially observed; subsequently, compared with the control, its concentration reduced by ∼2.8 log CFU/mL at an MOI of 100 (p⟨0.05). The overall bacterial reduction was associated with high MOI values of the phage. Then, bacterial regrowth sharply occurred until 6 h, and no significant differences were observed after 8 h (p>0.05). However, the bacterial reduction by ST phage was greater than that by other Salmonella phages, such as PS5 at an MOI of 100 (reduction by 1.77 log CFU/mL) (Duc et al., 2020), SFP10 at an MOI of 10,000 (reduction by 2.00 log CFU/mL) (Park et al., 2012), and fmb-p1 at an MOI of 1 (reduction by 2.49 log CFU/mL) (Wang et al., 2017). Thus, ST phage is a potential effective biocontrol agent as it can effectively control S. Typhimurium over a 2 h treatment period. Because regrowth occurred after the 2 h exposure, the antibiofilm potential of the ST phage was investigated within 2 h to ensure its performance on PP surface. Thus, the ideal phage treatment conditions in a PP tube were determined to be an MOI of 100 and 2 h exposure based on the most effective bactericidal effect of ST phage.
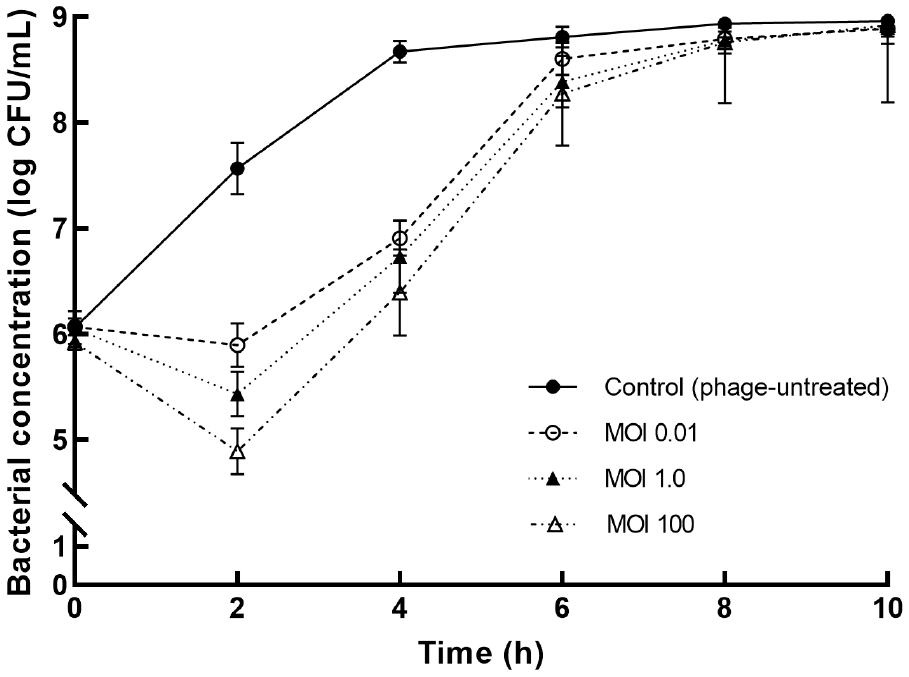
The antibiofilm potential of ST phage was evaluated based on biofilm biomass, as determined using CV staining (Fig. 4), and biofilm population, as estimated using the plate count method (Fig. 5). After incubation for 24 h, the formation of S. Typhimurium biofilm on PP surface was confirmed by CV staining. Based on the result of the bactericidal effect, ST phage was added at an MOI of 100 for the control of biofilm formed on PP surface. Compared to the control, the absorbance of CV-stained biofilm on PP surface significantly reduced until 90 min of phage treatment (p⟨0.05) (Fig. 4). No further increase was observed over the next 90 min, indicating no biofilm regrowth on PP surface. CV staining is one of the most common methods for biofilm quantification. It provides the total biofilm biomass as CV can stain both viable and dead cells in its matrix (Azeredo et al., 2017). Furthermore, viable S. Typhimurium cells in biofilm (biofilm population) were determined using the XLD plate count method (Fig. 5). S. Typhimurium biofilm formed and matured on the PP tube surface with a biofilm population of 6.9±0.15 log CFU/cm2. Subsequently, ST phage treatment significantly reduced the biofilm population on PP surface by 1.6 ±0.1 log CFU/mL at 30 min and 2.8±0.12 log CFU/mL at 60 min (p⟨0.05), and this reduction was sustained after 60 min.
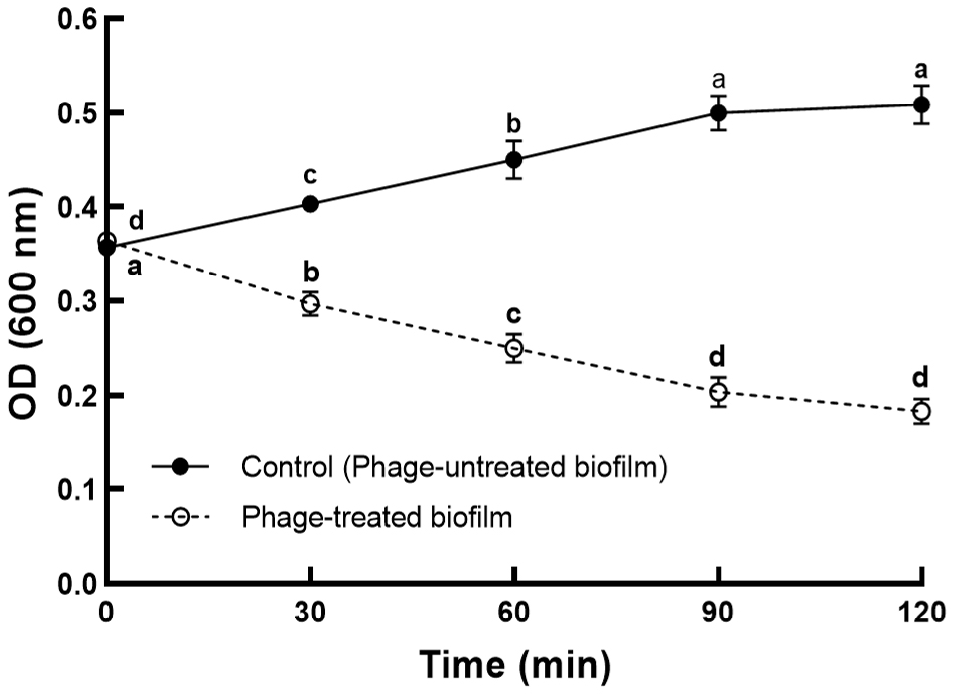
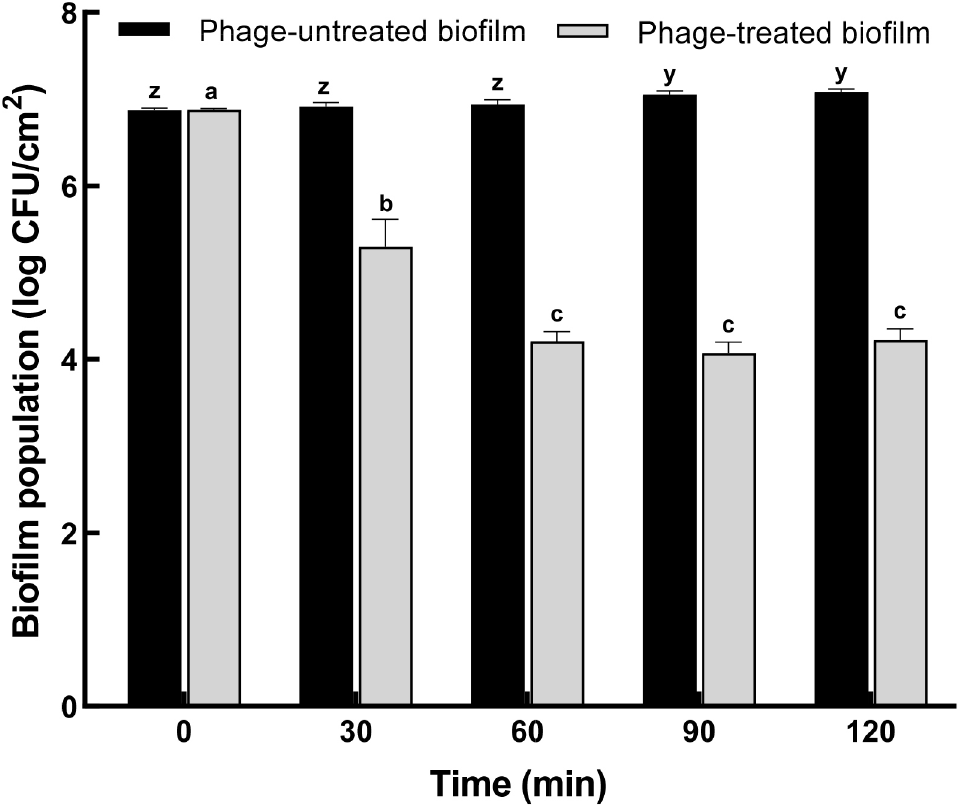
So far, only few studies have been reported on controlling Salmonella biofilm on food contact surfaces using Salmonella phages. As the absorbance values obtained following the CV staining method cannot be compared objectively, the biofilm inhibition rate was used to compare the efficacy of phage treatment among different studies. Our ST phage inhibited S. Typhimurium biofilm formation with a capability of ∼63% at an MOI of 100 after 2 h treatment (Fig. 4). A previous study (Islam et al., 2019) reported a comparable capability (44%-63%) of a phage cocktail comprising LPSTLL, LPST94, and LPST153 against S. Typhimurium biofilm on polystyrene plates. Another study (Kuźmińska-Bajor et al., 2022) reported that the phage cocktail of UPWr_S1, UPWr_S3, and UPWr_S4 exhibited high (∼98%) or comparable (∼69%) capabilities against S. Enteritidis biofilm on polystyrene or stainless steel, respectively, at an MOI of 10. Additionally, the antibiofilm potential (∼2.8 log CFU/cm2) of our phage was greater than that (>1 log CFU/cm2) of PVP-SE2 phage against S. Enteritidis biofilm on stainless steel (Milho et al., 2018) or was comparable to that (∼2-3 log CFU/cm2) of a phage cocktail (BP 1,369 and BP 1,370) against S. Typhimurium biofilm on stainless steel and rubber (Sadekuzzaman et al., 2018). These results suggest the sufficient antibiofilm potential of ST phage for use as a biocontrol agent in the food industry.
4. Conclusion
Several studies have demonstrated the antibacterial activity of Salmonella phages, however, their application for controlling Salmonella biofilm is limited. In this study, a lytic ST phage isolated from a slaughterhouse exhibited a bactericidal effect and antibiofilm capability. Therefore, ST phage can be used as an effective biocontrol agent in the food industry. Since the relationship between antibiofilm capability of phages and phage-derived enzymes (Chang et al., 2022), the future study will focus on the genetic characterization of ST phage to identify the existence of phage-derived depolymerase and/or endolysin.