1. Introduction
During the current COVID-19 crisis, a primary immune system to fight diseases is more strongly required than ever. Therefore the demand for functional fermented foods with physiological activities, such as disease prevention, treatment, or improvement, has been increasing. Vinegar, manufactured using acetic acid bacteria (AAB), has long been used to treat diseases in both the East and West (Kim et al., 2020). Acetic acid-producing bacteria form a bacterial film on the alcohol surface and ferment the alcohol to acetic acid. Bacterial cell bodies are dissolved in the manufactured product during the maturation process to produce rich vinegar. Since ancient times, vinegar has been considered to contain both the cell components and acetic acid. Physiologically active substances in vinegar, which are metabolites of the fermentation using AAB, maintain the balance of body fluids and improve immunity against various diseases (Jin and Pyo, 2017; Kim and Shin, 2014; Kim et al., 2015; Koyama et al., 2017; Park et al., 2014). However, it cannot be said that acetic acid or polyphenols, the main components of vinegar, have all the functional properties of vinegar. Therefore, it is believed that various types of polysaccharides or protein-bound polysaccharide derived from bacterial cell bodies present in vinegar can function as new active substances (Anguluri et al., 2022; Aramsangtienchai et al., 2020).
Sugar chains bound to glycoproteins or glycolipids on the cell surface are involved in life phenomena such as cell differentiation, information transfer, infection, and cancer metastasis through cell-to-cell recognition and adhesion (Paulson, 1989). Therefore, there has been a growing interest in the role of these polysaccharides in activating the immune system (Bao et al., 2002; Lee et al., 2014; Shin et al., 1997; Zhu et al., 2008). Lipopolysaccharides (LPS), cell wall components of Gram-negative bacteria, can activate innate immune responses after recognition by immune receptors. LPS immune activity plays a central role in the toll-like receptor/myeloid differentiation factor-2 (TLR4/MD-2) complex and glycolipid A. Glycolipid A anchors LPS to the outer membrane and activates the production of the pro-inflammatory cytokines tumor necrosis factor alpha (TNF-α), interleukin–1 beta (1L-1β), and interleukin-6 (IL-6) (Molinaro et al., 2015). LPS of E. coli is a hexa-acylated-bis-phosphorylated lipid A that has a strong affinity for the TLR4/MD-2 complex receptor and inducing a strong innate immune response. In contrast, hyperstimulation by functional LPS can release large-scale uncontrolled pro-inflammatory cytokines as it de-regulates innate immune system signaling. Therefore, modification of the lipid A structure can modulate and regulate innate immune responses, making studies on native LPS an important and interesting topic (Caroff and Karibian, 2003; Raetz and Whitfield, 2002). Hashimoto et al. (2016) reported that the ability of A. pasteurianus NBRC 3283 to be stable for a long time in an acidified environment is related to the lipid A structure of LPS. Interestingly, some lipid A showed weak or no immune activity (Lepper et al., 2005; Loppnow et al., 1990; Lorenzo et al., 2017; Pallach et al., 2018; Saitoh et al., 2004).
As part of the host’s defense mechanism, macrophages of the innate immune response recognize invasion of foreign substances and transmit immune information to lymphocytes. Activated macrophages produce nitric oxide (NO) and cytokines, and destroy cancer cells and harmful bacteria. Recently, many studies have reported the enhancement of immune actions with natural substances. Particularly, immune enhancers derived from natural substances are expected to enhance the immune response or restore the weakened immune function. However, only few studies have reported on the potential of AAB in this context. Immunopotentiating proofs of Amano et al. (2015) and Inagawa et al. (2019) were performed through oral administration of AAB to allergy suppression. Furthermore, Aramsangtienchai et al. (2020) showed immunomodulatory activity of AAB from the effect of sugar chains on NO production in macrophages cells. Therefore, we investigated the immune activation ability of AAB, the main producers of vinegar, which has been scarcely explored. Nuclear factor-kappa B (NF-κB) activation and production of NO and cytokines, TNF-α, IL-1β, and IL-6, were analyzed after treating the cell bodies of six A. pasteurianus into RAW-Blue cells. RAW-Blue™ cells derived from RAW 264.7 macrophages express a secreted embryonic alkaline phosphatase (SEAP) gene and awaken all TLRs induced by transcription factors of NF-κB and activator protein (AP)-1 (Haile et al., 2015). Therefore, we can easily detect and measure cellular activation for NF-κB and/or AP-1 leading to the secretion of SEAP into the cell supernatant through the activation of the macrophages by various antagonists.
In this study, we evaluated the immune response of six strains of AAB, which were isolated from farm-made fermented vinegars and recently characterized with excellent physiological functions, including antibacterial, antioxidant, antihypertensive and antidiabetic effects (Kim et al., 2022). We suggest that the expression of pro-inflammatory genes inducible NO synthase (iNOS), cyclooxygenase (COX)-2, TNF-α, IL-6, and IL-1β, regulated the production of NO and cytokines through AAB-stimulated NF-κB and AP-1 activation. Therefore, we report the useful biological activity as a functional ingredient of acetic acid bacterial cells through the immune enhancing function of A. pasteurianus.
2. Materials and methods
The six strains of AAB used in this study are : A. pasteurianus A11-2 (GHUR-A11-2, KACC 92203P) originating from vinegar of brown rice, A. pasteurianus A24 (SR-A24, KACC 92204P) originating from rice vinegar, A. pasteurianus A26 (GY-A26, KACC 92205P) originating from fruit vinegar of Yeongcheon, Gyeongbuk, Korea, A. pasteurianus A37 (GHF-37, KACC 92206P) originating from plum vinegar, A. pasteurianus B7 (JS-B7, KACC 92207P) originating from grain vinegar of Sunchang, Jeonbuk, Korea, and A. pasteurianus C1 (JS-C1, KACC 92208P) originating from fruit vinegar of Chuncheon, Gangwon, Korea. These strains were isolated, reported and stored in the Fermented Processed Foods Division of the National Academy of Agricultural Sciences (Kim et al., 2022). The AAB were cultured at 30°C using a solid (yeast extract 0.5%, glucose 3.0%, CaCO3 1.0%, agar 2.0%, and ethanol 3%, w/v) and liquid media (yeast extract 0.5%, glucose 0.5%, glycerol 1.0%, MgSO4․7H2O 0.02%, ethanol 4.0%, and acetic acid 1.0%, w/w).
RAW-Blue™ cells (InvivoGen, San Diego, LA, USA) used in this study were cultured at 37°C under 5% CO2 using Dulbecco’s modified eagle medium (DMEM, Gibco BRL Co., Grand Island, NY, USA) containing 10% fetal bovine serum (FBS, Gibco BRL Co.) and 1% penicillin-streptomycin (Gibco BRL Co.). To activate RAW-Blue™ cells, the cells were passaged once every 2 days, and they were cultured using DMEM medium supplemented with Zeocin (Gibco BRL Co.) once a week.
The viability of RAW-Blue™ cells was measured using the 3-(4,5-dimethylthiazole-2-yl)-2,5-diphenyl-tetrazolium bromide (MTT, Sigma-Aldrich Co., St. Louis, MO, USA) reduction method (Mosmann, 1983; Gil et al., 2018). RAW-Blue™ cells (1×105 cell/mL) were allowed to adhere overnight before they were stimulated with six strains of AAB (25, 50, 100 CFU/macrophage). The control group was treated with the same amount of media but were not stimulated by AAB. After stimulating for 24 h, 200 μL of MTT solution was added and the cells were continuously cultured for another 4 h. Incubation was stopped by adding 150 μL of dimethyl sulfoxide (DMSO, Sigma-Aldrich Co., St. Louis, MO, USA). Absorbance was measured at 540 nm using a microplate reader (SpectraMax M2, Winooski, VT, USA). The cell proliferation rate was expressed as a percentage (%) of the absorbance of each sample relative to the absorbance of control group.
RAW-Blue™ cells (1×105 cell/mL) were seeded in 96-well plates. Following 24 h of incubation to allow adherence, cells were stimulated with six AAB at each concentration (25, 50, 100 CFU/macrophage). NF-κB activation was determined after 20 h using the Quanti blue (InvivoGen, San Diego, LA, USA) assay. Briefly, through activation of cells using six AAB, NF-κB/AP-1 activation was induced, leading to secreted embryonic alkaline phosphatase (SEAP) into the cell supernatant. For detection, the cell supernatant and a SEAP detection reagent (Quanti blue) were mixed and absorbance was measured at 650 nm (Brasier, 2006). LPS (100 ng/mL) was used as a positive control (E. coli O111:B4, Sigma-Aldrich Co.), and an experimental medium was used as a negative control.
Nitrite (NO2−) concentration was measured via a color reaction using Griess reagent (Promega, Masison, WI, USA). After pre-incubation of RAW-Blue™ cells (1×105 cell/mL) for 16 h, six AAB (25, 50, 100 CFU/macrophage) or LPS (100 ng/mL, v/v) were added for 24 h. The nitrite in culture supernatants was measured by adding 100 μL of Griess reagent mixture (1% sulfanilic acid, 0.1% N-1-naphthyl-ethylenediamine dihydrochloride, 5% phosphoric acid) to 50 μL of samples at 540 nm using a microplate reader.
The levels of TNF-α, IL-1β, and IL-6 were assessed in supernatants of RAW-Blue™ cells (2.5×106 cell/mL) stimulated with six strains of AAB (25, 50, 100 CFU/macrophage) or LPS (100 ng/mL, v/v) using each ELISA kit (Invitogen, Themofisher Scientific In., Vienna, Austria) according to manufacturer’s instructions (Kim et al., 2005).
RAW-Blue™ cells (2.5×106 cell/mL) were cultured in a 10×10 cell culture plate and incubated overnight. Cells were induced with six strains of AAB (25, 50, 100 CFU/macrophage), with LPS (100 ng/mL, v/v) and an experimental medium was used as control. Following 24 h of stimulation, total RNA was extracted from the cells using an RNeasy Plus Mini Kit (Qiagen, Valencia, CA, USA), following the manufacturer’s protocol. The extracted RNA (2 μg/μL) was used to obtain first-strand cDNA in a final reaction volume of 20 μL using cDNA Synthesis Kit (Bio-Rad, Hercules, USA). cDNA (5 μL) diluted five times were added to the reaction mixture containing 4 μL of ddH2O, 10 μL of 2×SYBR Green Master Mix (Bio-Rad, Hercules, USA) and 0.5 μL of each primer. The primers are listed in Table 1. Amplification was performed at 56.9°C for 40 cycles in an iCycler (Bio-Rad Laboratories) and data were analyzed using the iCycler iQ5 optical system software (Bio-Rad Laboratories). The relative expression levels were calculated using the formula ΔCt = [Ct (target, untreated) - Ct (β-actin, untreated)] / [Ct (target, treated) - Ct (β-actin, treated)]. The fold change in mRNA expression (ΔCt) was shown as the difference in threshold cycles (Ct) of the gene of interest after normalizing each gene to β-actin expression and control sample.
For statistical analysis, three replications of the experiment were carried out for each measured value and the data reported as the mean±standard deviation (SD) with n=3. Statistical processing was verified using one-way analysis of variance using SAS (Statistical Analysis System, v7.1, SAS Institute, INC., Cary, NC, USA), and p<0.05 level in Duncan’s multiple range test was considered significant.
3. Results and discussion
First, the effect of the six AAB strains on macrophage proliferation was investigated through a tetrazolium-based colorimetric (MTT) assay. The 540 nm-absorbance is a method of measuring the degree of reduction of MTT tetrazolium, which is a yellow water-soluble substrate, to dark purple insoluble crystals of MTT formazan due to action of dehydrogenase in the mitochondria of living cells. It reflects the concentration of vigorous cells (George and John, 2006). RAW-Blue™ macrophages, treated with 25, 50, and 100 CFU/macrophage of six AAB strains, showing no toxicity to macrophages and increased cell proliferation rate by activating macrophages (Fig. 1). Therefore, the immune activity test was performed using three treatment concentrations (25, 50, 100 CFU/macrophage) of the cell bodies for six AAB strains.
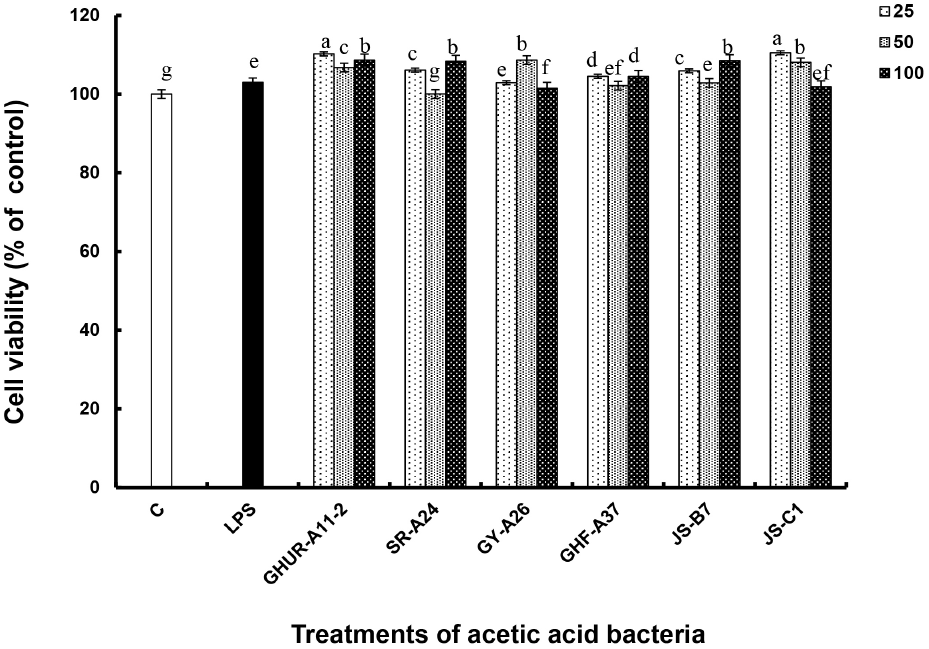
The immunostimulatory activities of six acetate strains of A. pasteurianus were investigated using RAW-Blue™ macrophages. NF-κB activates transcription factors of genes involved in immune responses to various stimulatory factors, including cytokines and toll-like receptor ligands such as LPS (Brasier, 2006; Hoffmann et al., 2006). The macrophage is an NF-κB/AP-1 reporter cell line derived from mouse RAW 264.7 macrophages. These macrophages contain the NF-κB/AP-1 inducible SEAP reporter gene, and they are activated when stimulated by an immune response. As a result, it was reviewed through the change from pink to blue by the indicator (Quanti blue). NF-κB activities of the six AAB strains were expressed as a fold value as compared to the activity generated by LPS (100 ng/mL, v/v) as a positive control (Fig. 2(A)). Except for GHF-A37, five AAB enhanced NF-κB activity in a concentration-dependent manner. Particularly, the activity of JS-C1 was as good as that of LPS treatment, and the rest of the strains had 7-8 times higher activity than the negative control.
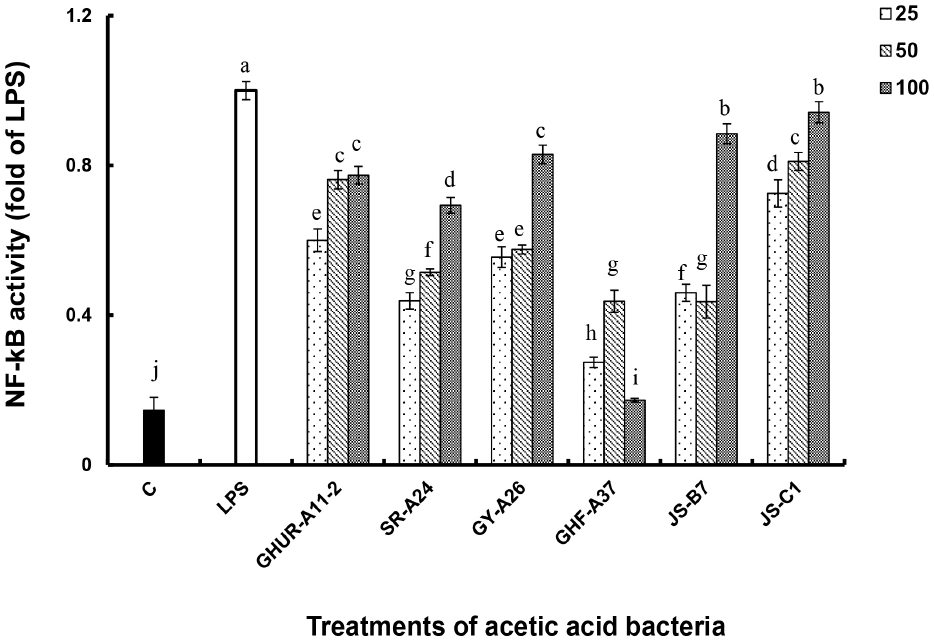
Antioxidant enzymes and antioxidants protect the living body. However, when abnormalities occur due to physical and chemical influences, reactive oxygen species (ROS) are generated, indicating oxidative stress as a defense system. With this mechanism, NO generated from exposure to toxic or harmful substances plays an important role in blood coagulation and blood pressure control functions as an indicator of inflammatory response and immune function against cancer cells (Gomes et al., 2005; Lim, 2004). The amount of NO produced by treating RAW-Blue™ cells with different concentrations of six AAB strains (25, 50, 100 CFU/macrophage) was compared to the amount of NO produced by the positive control LPS, which induces NO production in macrophages (Fig. 3).
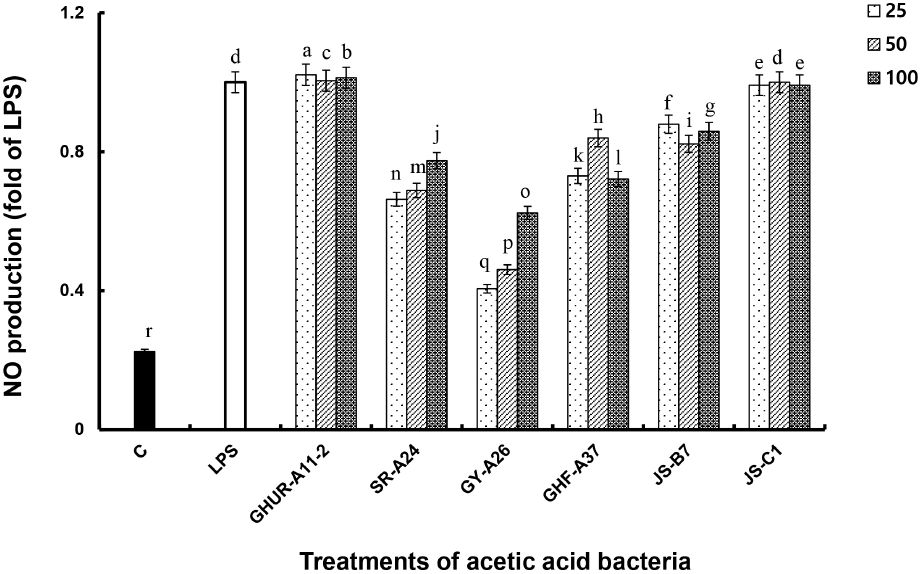
Regardless of the concentration of treated cells, AAB GHUR-A11-2 and JS-C1 generated NO at a higher or similar level to that generated by the LPS treatment. The NO production capacity of the AAB JS-B7 and GHF-A37 was 0.7-0.9 times higher than that of LPS treatment with a low concentration. The AAB SR-A24 and GY-A26 showed NO production in a concentration-dependent manner, with 2-4 times the effect as compared to the negative control group (medium). Macrophages play an important role in the immune system as part of the host’s defense mechanism. Macrophages activated by recognizing invasion of foreign substances can inhibit the growth of cancer cells and various harmful bacteria by enhancing their macrophage ability and generating NO and cytokines (Kim and Kang, 2008). The above results indicated that the six AAB strains could increase the immune function by activating macrophages, which increased NO production. Therefore, six AAB strains can be used in functional food as they have a favorable effect on immune response.
Substances (LPS or natural products) that respond to toll-like receptor (TLR) activate macrophages to produce cytokines such as TNF-α, IL-1β, and IL-6, which regulate secondary immune responses such as proliferation of T and B cells, activation of macrophages for phagocytosis, and defense against microbial infection (Lepper et al., 2005; Saitoh et al., 2004). TNF-α is an inflammation-mediated cytokine produced by lymphocytes, acting alone or in combination with cytokines, such as IL-1 in the in vivo immune response to damage tumor blood vessels, resulting in tumor necrosis or improving infection resistance by microorganisms (Visner et al., 1990). IL-6, which is a major reaction mediator generated in the initial immune response following infection and tissue damage, induces gene expression stimulated by TNF-α and promotes or inhibits rapid inflammatory response by monocytes, macrophages, and stromal cells (Hashimoto et al., 2016). Additionally, IL-1β is an active type of caspase-1, which is produced by macrophages activated by proproteins through proteolysis and plays an important role in mediators of inflammatory responses and in various cellular activities such as cell proliferation, differentiation, and apoptosis (Eltom et al., 2014).
Six AAB strains stimulated macrophages to induce TNF-α production and showed excellent activity even at relatively low concentrations. All AAB strains, except GY-A26, showed higher activity than the positive control (Fig. 4(A)). The GY-A26 showed 7-fold activity as compared to the negative control. In the case of IL-1β, all strains except GY-A26 showed a significant increase in activity in a concentration-dependent manner (Fig. 4(B)). In contrast, in the case of IL-6 production ability, the activity showed a tendency to increase in a concentration-dependent manner as compared to that of the negative control; however, it showed a relatively low activity than the positive control (Fig. 4(C)). Through these results, macrophages stimulated by six strains of AAB are thought to induce various immune stimuli by activating differential production of TNF-α, IL-1β, and IL-6. The production of these appropriate cytokines is thought to play an important role in regulating the immune system. Meanwhile, inflammation signaling involves multiple mechanisms. By interacting with their promoter regions, NF-κB modulates the expression of COX-2, iNOS, and other pro-inflammatory genes in LPS-stimulated macrophages. This necessitated the investigation of the expression of COX-2, iNOS, and pro-inflammatory genes in the immune activation ability of AAB.
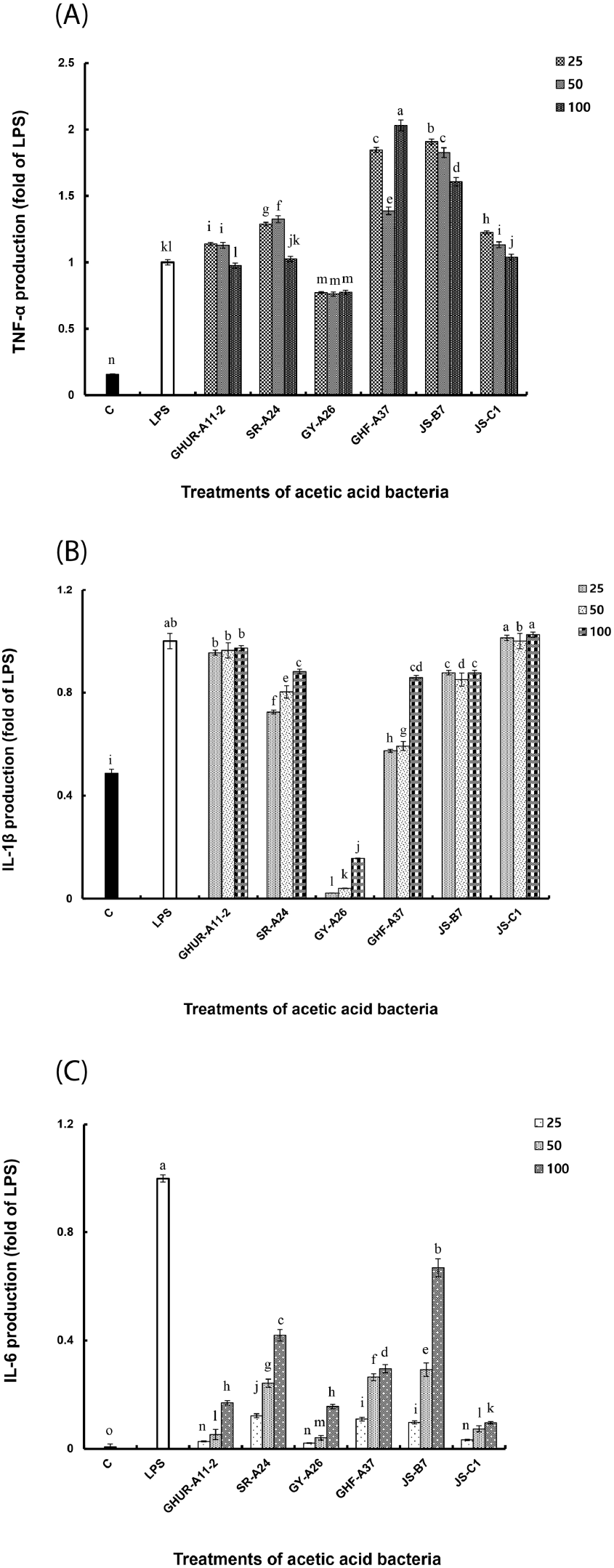
The effects of AAB on immune responses were mediated by activating the NF-κB/AP-1-JNK/p38 MAPK signaling pathways (Zhou et al., 2008). LPS activates all three MAPKs in macrophages (Guha and Mackman, 2001). Different MAPKs may play a role in the up-regulation of pro-inflammatory genes in LPS-stimulated macrophages. JNK can regulate iNOS and COX-2. NF-κB stimulates cytokine production, thereby playing an important role in macrophage activation. Furthermore, NF-κB can induce the transcription of genes encoding the cytokines TNF-α, IL-6, IL-1, and interferon (INF) (Baeuerle and Henkel, 1994; Ghosh et al., 1998).
To explore the underlying mechanism, we determined the mRNA expression of iNOS and COX-2 closely related to signal transduction of NF-κB/AP-1 activation (Fig. 5). Consistent with the above-mentioned reports, our results revealed that the AAB-stimulated iNOS and COX-2 expression induced NO and cytokines production, thereby suggesting that the immunomodulatory function exhibited by AAB may involve the activation of the NF-κB/AP-1 signaling pathways (Fig. 6). As a major transcription factor, NF-κB plays a key role in regulating genes responsible for innate and adaptive immune responses (Gil et al., 2018). Furthmore, these pro-inflammatory mediators and cytokines can lead to inflammation and various clinical manifestations. Overexpressed pro-inflammatory mediators further exacerbate immune responses in many acute and chronic inflammatory diseases. Considering these disadvantages, the six A. pasteurianus may be unhealthy. However, the effect of immune response of the AAB-stimulated macrophage examined in this study suggests AAB’s excellent potential as not only a functional food, but also important strains in the vinegar industry.
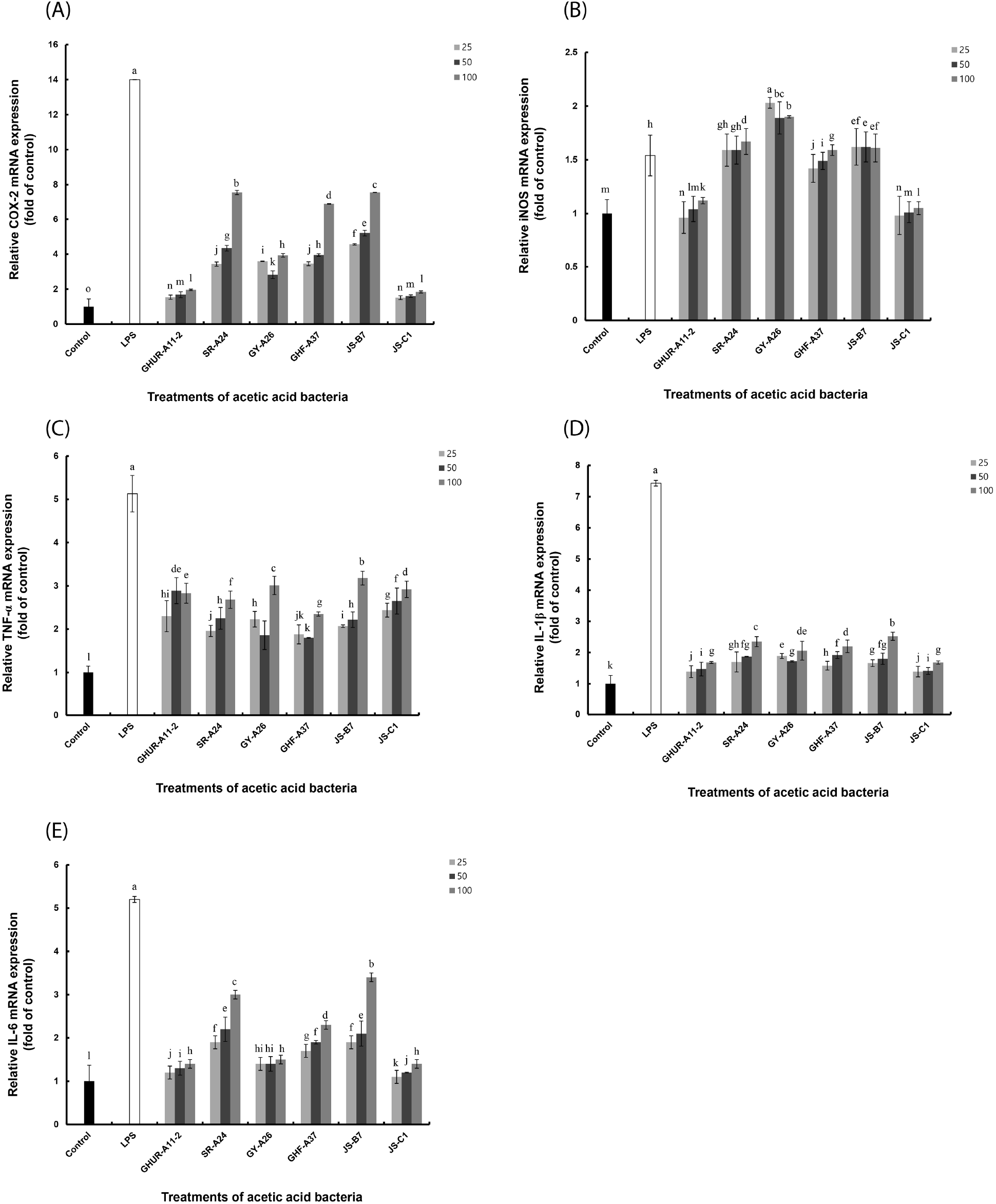
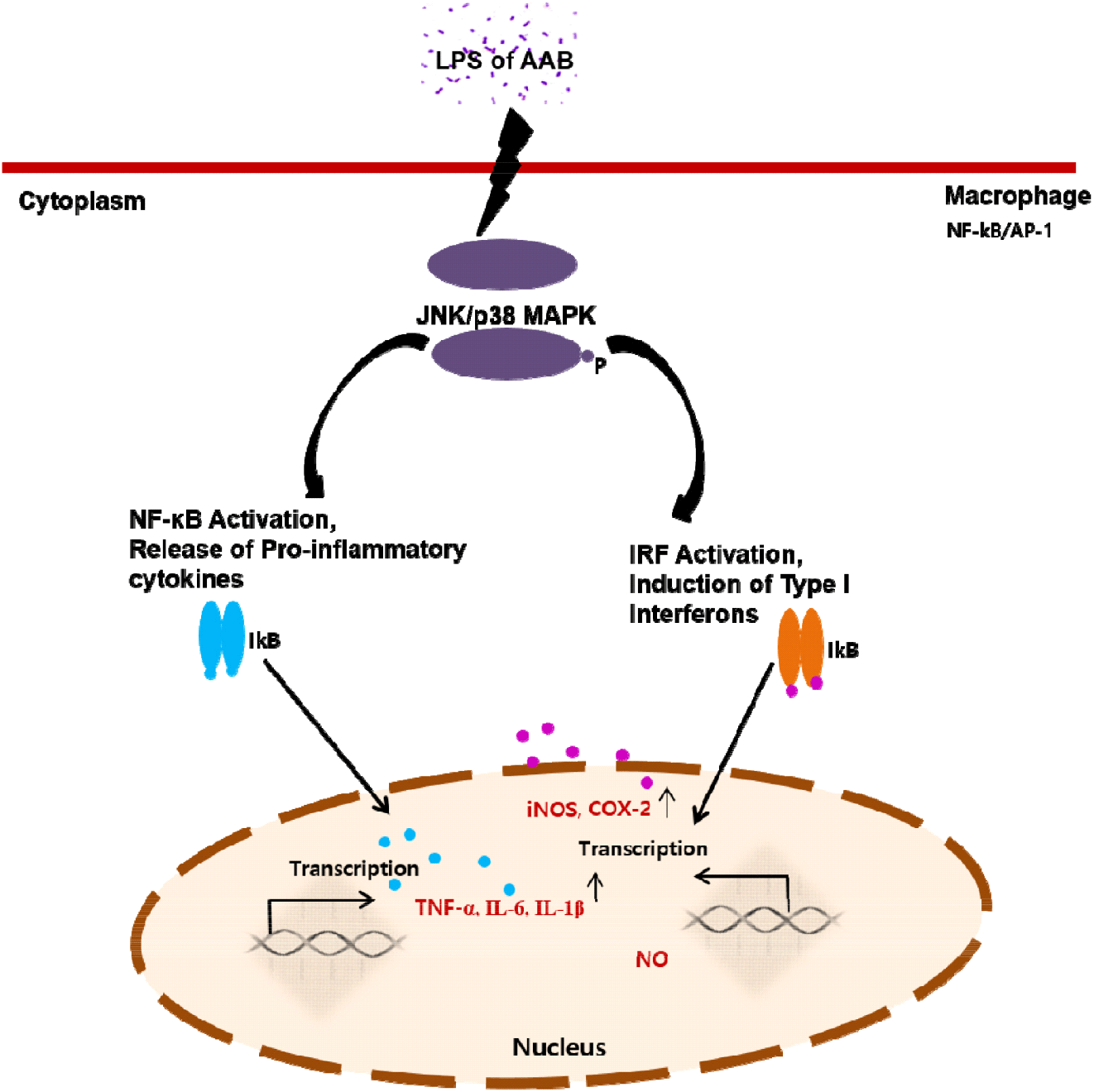
4. Conclusions
This study revealed the immunological properties of six A. pasteurianus strains through NF-κB/AP-1 transcription factor activation and NO and cytokine production in macrophages. The six strains have LPS that enhanced the ability of NF-κB/AP-1 to activate transcription factors. Total RNA and protein were extracted from cell to analyze the expression for pro-inflammatory cytokines COX-2 and iNOS, and their protein. The production of NO and cytokines (TNF-α, IL-1β, and IL-6) of the six strains was higher than that of the controls, with different immune activation abilities for each strain. These results suggested that the six strains of A. pasteurianus strains have an excellent industrial application potential in the vinegar industry and serve as functional foods.